PCBs are an essential component in electronic applications, making performance, reliability, and safety-critical factors to consider. To ensure these aspects, engineers must comprehend how temperature impacts the quality of their printed circuit board assembly during the development phase, even before PCB creation begins.
PCB design comprises several components like holes, traces, solder masks, layers, and through holes, each susceptible to heat. Elevated temperatures can have negative consequences on standard PCBs, as well as any other type of PCB. The PCB’s element determines its maximum temperature, known as the printed circuit board temperature limit.
What is the typical temperature range for PCBs? The temperature range of a PCB is measurable by the material, resulting in variations in the temperature limits for different PCBs.
FR-4 is the most prevalent substrate useful in PCBs, comprising a flame-resistant glass epoxy substrate. It can endure temperatures ranging from 90°C-110°C.
High-temperature printed circuit boards are typically categorized as those with the Tg exceeding 170°C, although some may use 150°C as the threshold. The specific definition may differ among high-temperature printed circuit board manufacturers.
How to measure PCB Water Temperature?
It is important to consider thermal expansion before checking the temperature range of your PCB. Temperature monitoring is critical for identifying hot-power areas and power-hungry ICs and making necessary adjustments or corrections early.
Ensuring that the CTE aligns crucial if your printed circuit board has greater than one layer. CTE measures the expansion of materials at high temperatures. The dielectric layers must have a similar CTE to the copper coatings. If they are not together, each layer will expand at multiple rates, which can cause serious issues.
Measuring PCB Water Temperature
Before determining the maximum operating temperature of your PCB, there are some factors that you should consider.
- No need to rewrite this sentence as it is already clear and concise.
- Ensure the printed circuit board temperature indicator is installing properly before checking the temperature.
- Locate the ground pins, which should be with the laminate of the primary heat source. These ground pins facilitate the transfer of around 60 to 65 percent of the generated heat to the temperature sensor. Since the ground pins are with the laminate, they also have a minimum thermal resistance than other components among the heat source and the sensor.
Measure PCB temperature
- Place a ground plane between the temperature sensors and the heat source to achieve thermal equilibrium.
- Join the ground pins of every temperature gauge to the ground plane of the heat source.
- Maintain proximity between the heat source & temperature sensors.
Acceptable temperature boost for a PCB trace
The mechanical stability of the base material of a PCB becomes compromised at the glass temperature (Tg). It is important to note that the printed circuit board’s Tg is less than its melting point.
Each PCB has a unique glass transition temperature (Tg) based on its substrate. When the operating temperature exceeds the glass transition temperature, the printed circuit board’s mechanical stability becomes compromised. PCBs with a greater Tg value have improved stability at elevated temperatures because their substrates offer good heat resistance, chemical stability, and mechanical stability.
It is recommended that the Tg value of your PCB should be 20°C-25°C greater than the anticipated functional temperature of the equipment you are designing for. For example, if your PCB’s Tg value is 130°C, the device’s functional temperature must be less than 110°C.
PCB Water Temperature copper traces
Copper traces generate heat due to power dissipation from I2R, where I is the current and R is the trace’s resistance. The heat is then dissipated through the base material by thermal conductivity, radiation, and convection. The PCB temperature will stabilize when the heating or cooling costs are equivalent.
The copper trace current capacity is influenced by various factors, including the highest temperature rise needed and the trace width.
The trace width of a PCB depends on various factors, including the resistance, current flow, and the maximum temperature increase that can be tolerated.
Usually, a temperature increase of up to 10°C above the average temperature of twenty-five-degree centigrade is acceptable. However, in some cases, a temperature rise of up to 20°C may be permitted if the PCB design and material allow it.
What happens if the PCB Water Temperature drift is too high?
If the temperature rises in your PCB’s copper trace is too high, such as 50°C or 100°C, it can negatively impact nearby components’ ambient temperature, leading to decreased board integrity and power loss. While a copper trace on a FR 4 board may be able to handle such a temperature rise, it’s still important to consider the potential consequences.
Common causes of PCB Water Temperature increases
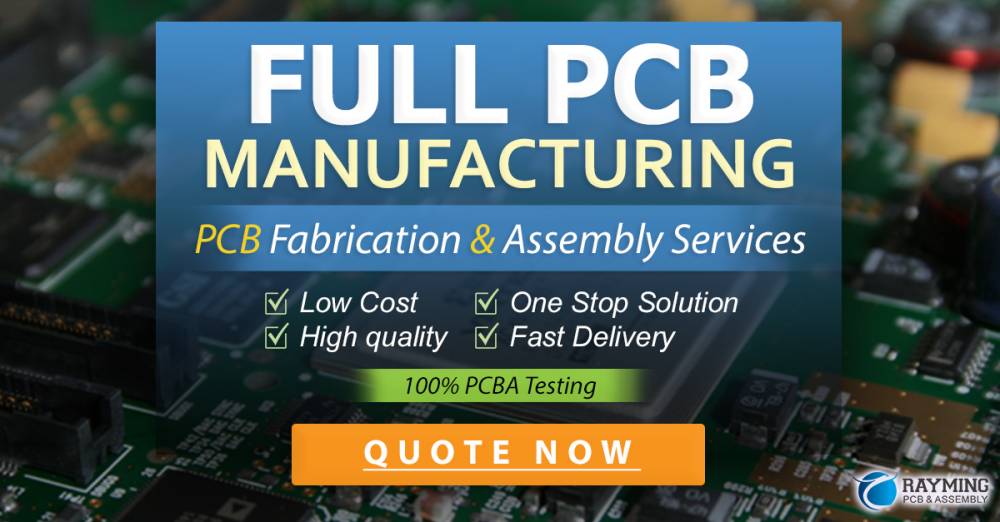
There are several reasons why PCBs may experience high temperatures; some of the most usual include:
· Malfunction causes heat dissipation
If a component malfunctions on your printed circuit board, it can lead to heat dissipation. The faulty component can no longer create high power, which causes nearby components to work more to compensate and generate more power. This results in the generation of more heat.
· Through-hole interference
Through-holes provide electrical connections among the layers through electroplating in the plated holes. The heat sink elements and through-holes are responsible for the power supply. However, if the heat dissipator doesn’t connect properly or if there is any obstruction with the PTH due to other components, it causes nearby elements to work harder, leading to the generation of more heat. This, in turn, leads to the maximum temperature.
· Lead-free solder
The use of lead in electronic applications is a hazardous material and increases challenges to health and environmental safety. As a result, design engineers globally comply with the EU’s RoHS by limiting the use of lead solder. However, the lead-free alternatives often have a higher melting point, resulting in PCBs requiring higher temperatures to operate, leading to increased stress on components.
· The distance of your surface mount
Proper distance between through-hole components and surface mount devices (SMDs) ensures a smooth current flow via a through-hole and heatsink. If placed too far apart, the current has to travel farther, resulting in cooling time for the receiving components. This can cause other components on the circuit board to create more power to compensate, ultimately leading to overheating.
· External thermal issues
It is important to consider the operating environment of the PCB and its components during the design phase. Neglecting this can lead to thermal stresses on the board in both high-temperature environments and extremely low. Resistors, capacitors, and semiconductors are among the components that can be affected by temperature changes.
· High-frequency circuits
High-frequency circuits are prevalent in mobile, microwave, high-speed design applications, and radio frequencies like smartphones. However, the downside is that these circuits tend to produce more heat due to the power they require. As a result, higher temperatures are inevitable in such boards.
There are several common methods for preventing temperature enhancement in PCBs.
To ensure high-performance PCBs, it’s essential to control heat through effective design. If the circuit board will be exposed to extreme temperatures, such as high or low environments, it’s crucial to consider this during the design phase. Follow these tips to maintain the optimal temperature of your PCB.
· Use heat sinks
Effective heat conductivity is crucial in controlling heat for high-performance PCB design. Heat sinks, typically made of aluminum or copper due to their outstanding thermal performance and low thermal resistance, are attached to components such as high-power transistors on the board to absorb and disperse heat in the air. The heat generated by the components is naturally transferred to the heat sinks.
· Choosing the right materials and components
Effective PCB design relies on using high-temperature-resistant materials. PCBs with thick copper plates are capable of withstanding high temperatures, which can extend the lifespan of the application. In contrast to conventional PCBs, these boards can handle elevated current levels, resist high temperatures for longer periods, and provide stronger connection features. The most commonly used material for PCBs is FR4, which is a glass epoxy that can tolerate high temperatures. Additionally, high-frequency elements like PCB laminates can provide outstanding dielectric attributes at various frequencies. When selecting a laminate, it is important to choose one that can absorb moisture and withstand high temperatures.
· Adequate Spacing
To prevent any interaction between the segments on your circuits, it is important to ensure sufficient space between them. Failure to do so could result in heat generation caused by the interaction between the components. By maintaining a distance between them, such issues can be avoided.
Minimum Temperature For Fr-4 PCBs
FR-4 PCBs typically endure temperatures as low as -50°C, beyond which brittle cracks may develop in the element. However, it’s advisable to avoid subjecting your FR-4 PCBs to extreme temperatures to reduce stress and extend their lifespan. In industries like aerospace, where PCBs may be deployed to outer space, they may be exposed to temperatures as low as -150°C, well below the recommended limit for the FR-4 printed circuit board.
Applications Of PCB Water Temperature
Thankfully, one can use several alternative materials instead of FR-4 to fabricate PCBs that require high-temperature resistance. One such material is polyimide, which can withstand a wide range of temperatures, including cryogenic conditions and temperatures as high as 260 Celsius.
Additionally, polyimide materials are highly resistant to fire. While polyimide materials offer excellent temperature resistance, they are vulnerable to water, making them a crucial factor to consider during the design phase.
Ceramic PCBs, on the other hand, are a more expensive option that offers the advantages of both worlds. These boards can withstand extreme temperatures, both low and high, and are impervious to water.
However, the high cost and design constraints with ceramic circuit boards are their downsides. In conclusion, there are meaningful differences or benefits among ceramic printed circuit boards. One should evaluate FR-4 circuit boards & MCPCBs evaluated for each application.
Due to its exceptional conductivity at or -272°C, aluminum holds great value in cryogenic printed circuit boards. However, the drawback of using aluminum is its high reactivity, which necessitates unconventional techniques for constructing circuit boards.