PCB stands for Printed Circuit Board. A PCB is a board used to mechanically support and electrically connect electronic components using conductive pathways or traces etched from copper sheets laminated onto a non-conductive substrate. PCBs are the foundation of almost every electronic device, allowing different components like integrated circuits, resistors, capacitors, and others to be interconnected and work together. Understanding what PCB means helps appreciate the important role PCBs play in electronics and how their design directly impacts the functionality, performance, and reliability of electronic devices.
What Does PCB Stand For?
PCB stands for Printed Circuit Board. Other names for PCBs include printed wiring boards (PWBs) and etched wiring boards. Some key points on what PCB means:
- PCB refers to the circuit board substrate made from an insulating material like FR-4 fiberglass, on which copper tracks are printed or etched to form the electrical connections between components.
- The “printed” part of PCB refers to the pattern of copper tracks or circuitry etched on the board which replaces individual wires. This pattern is created through methods like screen printing or photolithography.
- The use of the word “printed” does not mean PCBs are created by mechanically printing or stamping the wires onto the board. The patterns are painstakingly etched or removed from the copper layer bonded to the substrate.
- PCB allows the mounting of electronic components like ICs, resistors, capacitors, CPUs etc. which are soldered onto the copper tracks to complete an electronic circuit.
- Without a PCB, hundreds or thousands of individual wire connections would need to be made between components. The printed board provides organized, optimized, reliable connectivity.
In summary, PCB refers to the copper-laminated non-conductive board that provides the structural base and electrically conductive pathways to connect and power electronic components in a circuit. The board is etched with an intricate “printed” pattern of wiring traces substituted for individual wires. This technique revolutionized circuit assembly, enabling automated, reliable, miniaturized electronics.
A Brief History of PCBs
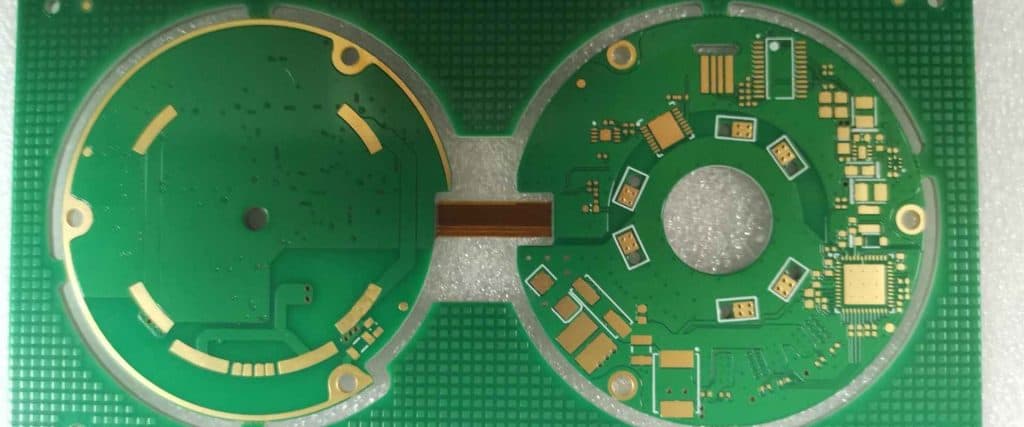
The earliest predecessor of printed circuit boards dates back to the early 20th century. Here are some key milestones in the history and evolution of PCBs:
- Early 1900s – Electricians soldered conductors like copper rods or strips directly to panel boards to assemble circuits and terminal equipment. This was labor-intensive, requiring individual wires to interconnect components.
- 1925 – Techniques emerged to assemble multiple terminal connections by machining grooves into a Bakelite wood-flour composite panel board, into which metal strips were inserted and then soldered.
- 1936 – The first actual printed wiring board using a precursor of today’s PCB was designed by Paul Eisler. He etched circuits onto Bakelite plates using conductive materials and photomechanical etching.
- 1940s – The US military began using printed wiring boards for bomb detonators and anti-aircraft proximity fuses during World War II.
- 1950s – PCBs were produced commercially and became the foundation of circuit assembly for electronic devices. FR-4 glass-reinforced epoxy laminate became the most common PCB substrate.
- 1970s – Advances in electronics manufacturing allowed greater circuit densities, trace sizes, and automation in PCB fabrication and component assembly.
- 1980s – Surface-mount technology enabled miniaturized surface-mount components to be soldered directly onto PCBs rather than using wire leads.
- Today – High-density multi-layer PCBs with elaborate cooling, shielding, and signal integrity enhancements are the backbone of cutting-edge electronics. New materials like ceramics and flexible substrates also expand PCB capabilities.
PCBs have evolved enormously, but still serve the same vital purpose – providing a structurally sound foundation to electromechanically support and connect electronic components using etched conductive copper pathways.
What is a PCB Made of?
A PCB consists of an insulating substrate reinforced with epoxy resin, onto which a layer of copper is laminated on one or both sides to create the conductive pathways between components. Here are the main constituent materials of a typical rigid PCB:
- Substrate – Usually a glass reinforced epoxy laminate sheet called FR-4. Other options include composites like FR-1 paper-phenolic and flexible substrates like polyimide.
- Conductors – Typically a thin sheet of copper foil (1 oz/ft2 or 35 μm) laminated to one or both sides of the substrate. Other conductor options include aluminum and silver.
- Dielectric Layer – The epoxy resin prepreg layer that bonds the conductive foils to the core substrate and insulates copper layers in a multilayer PCB. Common prepregs include FR-4 and polyimide.
- Finish – Coatings applied on top of conductors to protect and facilitate soldering. Common finishes include organic solderability preservatives (OSP), immersion tin, immersion silver, gold plating.
- Silkscreen – Epoxy ink printed on the PCB to label components and polarities, usually white or yellow.
- Solder Mask – Epoxy layer that coats the PCB covering copper traces, exposing only the solder pads. Usually green color.
- Drilled Holes – Holes drilled through the PCB substrate to allow leads or wires to pass through and connect layers. Plated with copper.
While FR-4 fiberglass is the most common, a wide range of substrate materials like Rogers, polyimide, Teflon, and others can be used. Different materials provide various benefits in performance, temperature tolerance, flexibility, volume manufacture, and cost.
How is a PCB Fabricated?
PCBs are fabricated using the following general sequence of steps:
- Design – The PCB layout is designed in software like Eagle, Altium or KiCad. The schematic and board outlines are drawn.
- Prototyping – For simple circuits or testing, DIY methods like milling or toner transfer can create single PCBs.
- Panelization – For volume production, the PCB design is replicated in a panel for mass lamination.
- Lamination – Sheets of copper-clad substrate are stacked with prepreg dielectric layers and laminated under heat and pressure.
- Drilling – Holes are precision-drilled for component leads using small drill bits, often controlled by a CNC drill machine.
- Plating – Walls of drilled holes are plated with copper to electrically connect layers of the PCB.
- Etching – Unwanted copper is selectively removed by chemical etching to leave only the desired conductive copper traces on the substrate.
- Solder mask – UV-curable epoxy layer is applied by spraying, screen printing or photo-imageable process.
- Silkscreen – Component outlines and identifiers are screen printed on the solder mask ink.
- Routing – Individual PCBs are routed or cut from the larger panelized boards.
- Testing – Quality checks are performed, including electrical testing, visual inspection, and x-ray.
- Assembly – Electronic components are soldered onto the fabricated PCB.
The specific fabrication steps and sequence may vary across different PCB manufacturers and for specialized PCB technologies. But this gives a general idea of the PCB fabrication process.
PCB Classifications
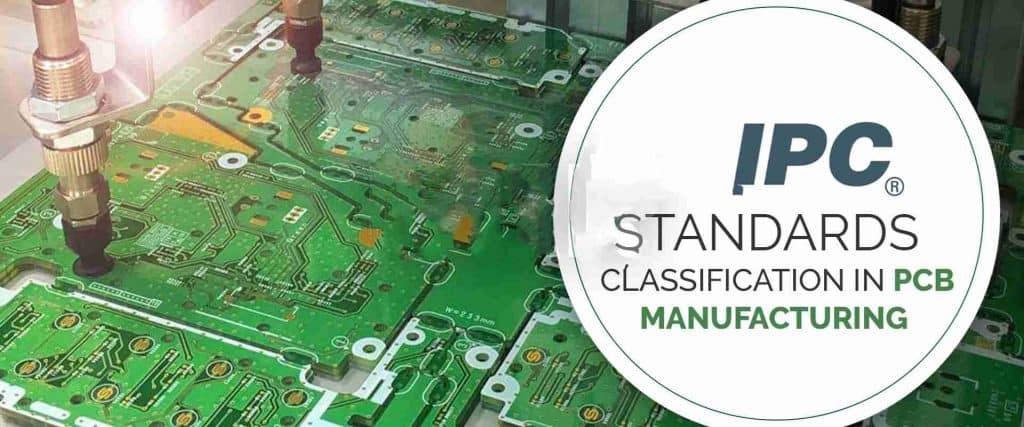
PCBs can be classified based on the number of conductive copper layers or “sides” as follows:
Single-sided
- One copper layer, usually on top side of substrate. Components mounted on same side.
Double-sided
- Two copper layers, one on each side of substrate. Allows increased component density.
Multilayer
- Has three or more copper layers laminated with insulating dielectric, interconnected by plated through-holes. Allows greater complexity.
- Most common are 4-layer and 6-layer, but can go up to 32 layers or more.
- Outer two conductive layers are for component mounting. Inner layers are for power distribution and signal traces.
In addition to layer count, PCBs can also be classified by conductive pattern fabrication technique:
- Printed Circuit Boards (PCB) – Circuits etched from copper laminated on substrate.
- Printed Wiring Boards (PWB) – Wiring traces are printed by plating into a blank pattern.
As well as types of substrate materials:
- FR-4 Boards – Most common. Made from glass reinforced epoxy resin.
- Flexible Circuits – Made from polyimide, flex PCBs can bend and twist. Used for wearables or space-constrained devices.
- Metal Core PCBs (MCPCB) – Aluminum or copper substrate removes heat from power components.
- High-frequency Boards – Use low-loss dielectric substrates for RF/microwave circuits.
- Ceramic Boards – Used for robust high-temperature automotive or aerospace electronics.
And there are many other classifications as PCB technology continues advancing. But layer count, fabrication process, and base substrate are the main categories for differentiating PCB types.
PCB Design and Layout
Creating the physical layout and wiring plan for a PCB is a skilled task mixing engineering design with software CAD tools. Here is a simplified overview of the PCB design and layout process:
Schematic Capture
- The electronic circuit is mapped out in schematic diagram form using CAD software like Eagle, OrCAD, Altium, KiCAD etc.
- Symbols represent each component and lines between them represent conductive connections.
- The schematic captures the logical electronic functionality without indicating physical position.
Floorplanning
- The physical board dimensions and component placement is determined based on parameters like desired device size, shape, component sizes, and required clearances.
- Critical traces may be mapped out beforehand between points where key components must connect.
Layout
- Components are arranged and routed on the board canvas. CAD tools allow the layout to be flipped and rotated while visualizing trace clearance.
- Traces are neatly laid out connecting nodes between components following established routing conventions like 45-degree angles and minimizing crossovers.
- Simulation checks can identify issues like electrical noise, signal reflections, power delivery problems, or electromagnetic interference that may impact performance.
- The designer ensures specifications are met and design rules adhered to. Numerous adjustments are made iteratively to optimize the layout.
Final Output
- Manufacturing files like Gerber files, drill files, and IPC-356 testpoint report are generated. Many output file types are needed by PCB fabricators.
- Final design validation is done before releasing to fabrication.
While simplified, this captures the progression from functional schematic to physical PCB layout while balancing electrical performance, manufacturability, and cost constraints.
Applications and Advantages of Using a PCB
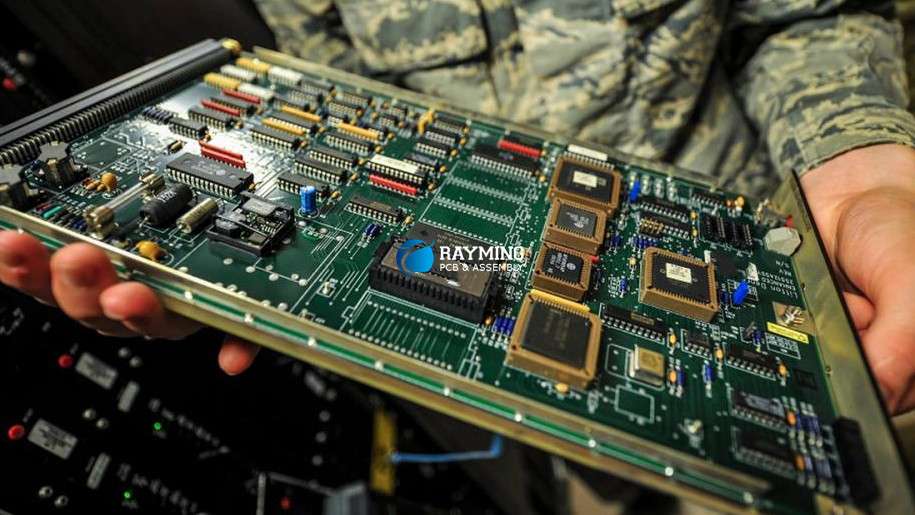
Here are some of the key applications and benefits that make PCBs an indispensable technology across nearly all modern electronics:
- Circuit Miniaturization – PCBs allow extremely small and complex circuits to be reliably mass-produced – a feat impossible with individually assembled wires.
- Organized Layout – PCBs allow optimized component placement and routing to minimize electrical noise and enhance performance.
- Cost Efficiency – Automated PCB production is extremely cost-effective compared to manual wiring and soldering.
- Reliability – PCB assembly eliminates wiring errors and loose connections prone with point-to-point wiring.
- Serviceability – Individual failed components can be desoldered and replaced quickly.
- Multi-layer Circuits – Multilayer PCBs allow complex, high-density interconnections between components.
- Component Mounting – PCBs provide firm mechanical support and electrical terminals for mounting components like ICs, resistors, connectors etc.
- Ease of Assembly – Automated PCB assembly, soldering and test processes can be implemented with proper design.
- Heat Sinking – Some PCB substrates like MCPCBs and ceramics excel at conducting heat from high-power components.
- System Integration – PCBs allow integration of circuits, microprocessors, memory, sensors and other technologies into a unified system.
From the integrated circuits powering supercomputers to LED throwie art projects, PCBs enable nearly all modern electronic devices. Correct PCB design ensures the device performs properly, reliably, and safely.
Common PCB Terminology
There are many industry terms associated with PCBs that are good to know:
- Traces – The copper lines that form the electrical connections between points on a PCB. Also called tracks.
- Pads – Metallic areas on the PCB where component leads or wires are soldered to traces.
- Plated-through Hole (PTH) – Holes drilled in a multilayer PCB with metal plating to interconnect layers. Also called vias.
- Annular Ring – Ring of clear space around a drilled hole, important to ensure reliable plating.
- Jumper – Short trace “jumping” over a trace or component to connect two points without intersecting.
- Plane – Large copper area used for power distribution or heat dissipation.
- Breakout Board – Small board that connects headers to various individual pinouts or ports as a transition to cable connectors.
- Keepout – Area of bare board that must be kept clear of copper and components, usually for clearance. Denoted on silkscreen.
- Tenting Vias – Covering the top and bottom of a via with solder mask to prevent solder from plugging via barrel during reflow.
- Castellated Holes – Half-drilled plated holes along the board edge allows surface mount soldering to external connectors or daughterboards.
And many additional terms for via types, hole shapes, trace widths, junctions, lands, thermal relief, testpoints and other PCB features. The expansive terminology reflects the extensive engineering considerations made in PCB design.
Key Factors in PCB Design
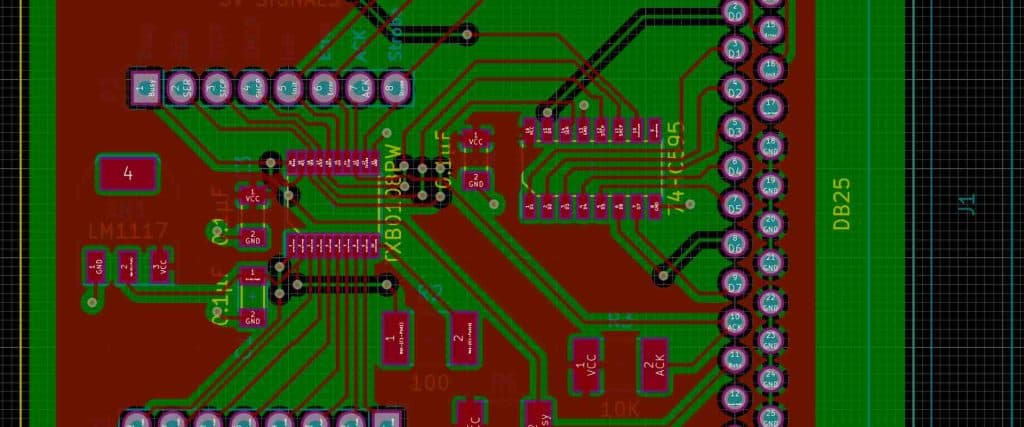
Designing a functional, reliable printed circuit board requires careful consideration of electrical, thermal, and mechanical factors:
- Circuit Function – Does the PCB schematic and layout accurately produce the intended behaviors of the target electronic circuit or system? Verification requires testing and simulation.
- Trace Current – Track widths must be sized appropriately for the expected current flows to avoid overheating and maintain signal integrity.
- Impedance Control – Matching trace impedances to terminal impedances prevents signal reflections and unintended inductances.
- Noise Reduction – Careful routing and ground planes suppress electrical noise and crosstalk, especially at high frequencies.
- Power Distribution – Power and return paths must be adequate for all component power needs. Planes help here.
- Thermal Loads – Copper planes or thermal vias help conduct heat out of high power components.
- Mechanical Strength – Materials and stackup must provide sufficient strength, especially where connectors attach.
- Manufacturability – Design must adhere to fabricator capabilities and domain-specific guidelines like IPC standards.
- Testability – Incorporating testpoints and vias for probing aids defect analysis and troubleshooting.
The PCB designer has responsibility for considering all these aspects and more to create a functional board that can be economically manufactured and works reliabily.
PCB Design Software
There are many PCB design software options at a range of prices, features, and complexity:
Software | Description |
---|---|
Eagle | Popular entry-level tool owned by Autodesk. Available as free version with limited board size. |
KiCad | Full open-source suite with schematic capture, routing, and 3D viewing. Steep learning curve but very powerful. |
Altium Designer | Industry-leading tool with unified design environment. High cost but advanced features. |
OrCAD | Full PCB design solution from Cadence with tight integrations between schematic, layout, and analysis tools. |
DipTrace | Affordable tool especially good for multi-layer boards. Downsides are performance issues at larger board sizes. |
EasyEDA | Popular online entry-level tool with free version. Limited feature set compared to downloads. |
Fritzing | Open-source tool focused on artists, students, and hobbyists. Breadboard sketching and hardware interaction are strengths. |
Multisim | Circuit simulation tool useful during schematic design to verify electronic behaviors before layout. |
And there are many other options. Key considerations when selecting PCB design software include cost, features, usability, output file generation, simulation/analysis capabilities, manufacturer integrations, and whether online or desktop install.
Recent Advances in PCB Technology
Some leading-edge developments in PCB tech:
- High-Density Interconnects (HDI) – Very dense trace routing and microvias, reducing layer count. Used in advanced consumer electronics.
- Embedded Components – Capacitors, resistors and other discrete passives buried inside the PCB layers. Saves space and cost.
- Flex and Rigid-Flex – Allows integrating rigid and flexible polyimide boards. Useful for wearables and compact folding.
- Stretchable Circuits – Novel materials and manufacturing methods now allow stretchable, flexible PCBs.
- Panel Plating – Plating internal layers and drilling vias while the PCBs are still in panel form for efficiency.
- Additive Processes – Additive deposition methods like inkjet and aerosol printing can directly “print” conductive traces, avoiding etching.
- RF/Microwave – Improved modeling and materials enable higher frequency boards above 3GHz. Key for communications.
- Automated Assembly – Emerging “lights out” production lines with AI-driven machines to enable highly automated PCB production.
And many other recent improvements allow PCBs to drive performance and integration in cutting-edge tech like IoT, EVs, 5G, and mobile devices.