An integrated circuit (IC) is a tiny collection of electronic components and circuits that have been implanted or diffused onto the surface of a single silicon chip or crystal. Because all parts, circuits, and base material come from one piece of silicon, the device is an integrated circuit.
The complexity of ICs ranges from basic logic modules and amplifiers to full-fledged microcomputers with millions of components. They have replaced vacuum tubes and transistors as the main building blocks of practically all electronic devices thanks to their low cost, excellent durability, low power consumption, and fast processing speeds. Although hydraulic, pneumatic, or mechanical controls were traditionally ideal, integrated circuit microcomputers are now helpful as equipment controllers, machine tools, vehicle operating systems, and other applications.
We may also update them without requiring the control circuitry to be changed. Finally, they are so cheap that they may even in electronic toys for kids.
Materials used in manufacturing integrated circuits
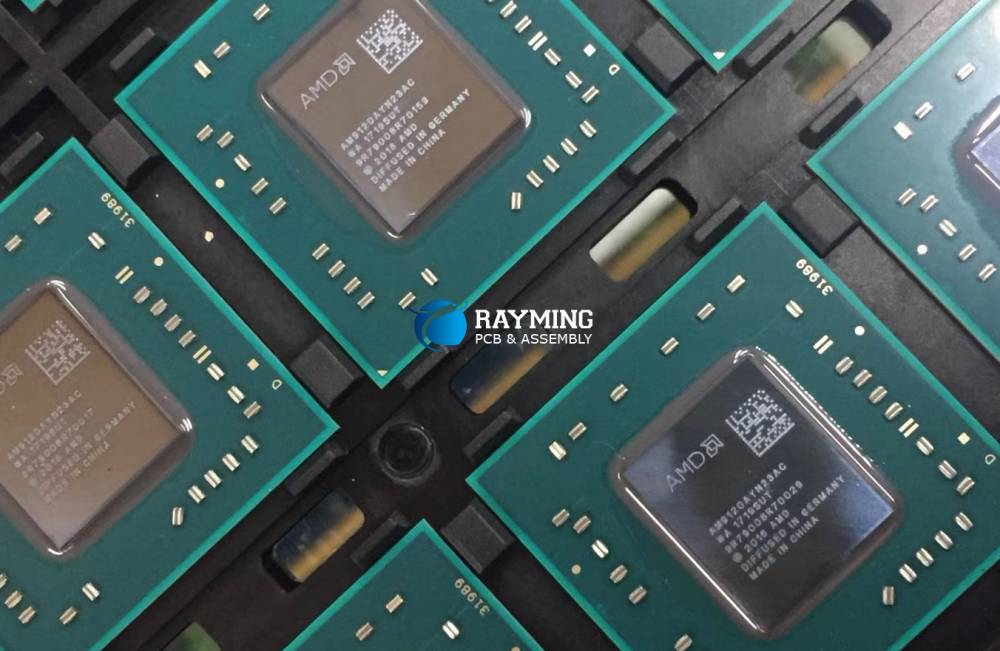
Silicon (Si) is the foundation of most modern electronics, powering everything from supercomputers to smartphones to air microwave ovens. It is an essential element because it is a semiconductor, which means that under certain conditions, it conducts electricity while acting as an insulator under others. Doping may change the electrical characteristics of silicon, making it a suitable material for building transistors that amplify electrical impulses. It is also a plentiful element on the planet, making it both economical and desirable. Gallium Nitride (GaN), which can work at higher voltages and is more tempting for high-speed high-power transistors for wireless applications, is not the sole element helpful in electronic devices.
Engineers have constantly found methods to improve silicon devices. Thus the benefits of utilizing silicon appear to expand year after year. Most of the electrical equipment you use daily would not be conceivable without silicon and silicon wafers.
Fabrication Techniques
An integrated circuit is normally created by a series of processes, each adding or removing material layers to achieve the required structure. The complementary metal-oxide-semiconductor (CMOS) process, which uses p-type and n-type silicon, is the most widely used manufacturing method for ICs.
A substrate, a thin silicon slice that acts as the IC’s base, is made as the first stage in the CMOS process. Monocrystalline silicon, a very pure silicon, is often used as the substrate.
After substrate preparation, we apply silicon dioxide (SiO2) as a layer on top. This layer acts as an insulator by blocking the passage of electrical current between various IC components.
After that, we decorate the silicon dioxide layer with designs using photolithography. This procedure exposes certain layer regions to UV light using a mask. Finally, we can remove the unexposed parts using a chemical etchant because the exposed areas become less resistant to chemical etching.
After making the designs, we dope the silicon to introduce impurities. Doping intentionally adds trace quantities of other substances to silicon, such as boron or phosphorus. Under specific circumstances, these impurities change the silicon’s electrical characteristics, allowing it to conduct electricity.
The silicon dioxide layer will make metal connections as the process’ follows the stage. Several IC components may send and receive electrical impulses thanks to these connections. Sputtering is commonly helpful to deposit a thin metal layer, such as aluminum or copper, on top of the silicon dioxide layer. The appropriate contacts are subsequently patterned onto the metal layer using photolithography.
The IC is then covered with a passivation layer to shield it from external elements, including moisture and mechanical stress. Often, silicon dioxide or silicon nitride is essential in creating this layer.
The manufacturing process of integrated circuits
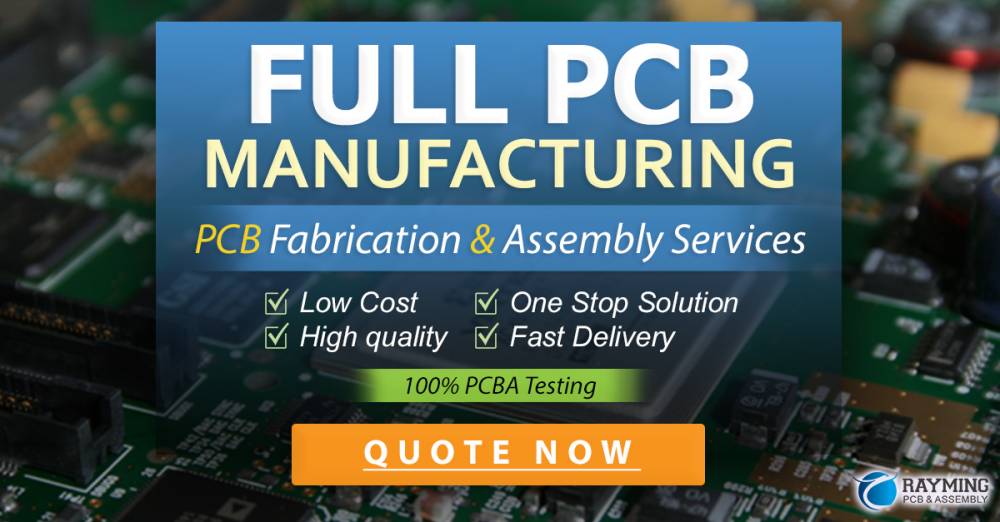
Creating many layers of materials on a substrate to create a functioning electronic device is a complicated procedure for fabricating integrated circuits (ICs). The procedure might take a few weeks and calls for specialized tools, sterile rooms, and qualified workers.
Wafer Preparation
A crucial procedure in the production of integrated circuits is wafer preparation. It entails several steps to create a smooth, clean, and defect-free surface on a silicon wafer. A silicon crystal must first grow before being sawed into thin wafers with a diamond saw. We then carry out the process of wafer lapping using a device that uses a spinning abrasive pad to scrape the wafer’s surface. The final step in producing a mirror-like quality is chemical mechanical polishing (CMP), which removes any last-minute surface flaws.
Combining wet and dry cleaning techniques helps to clean wafers. Before being dispatched for additional processing, we dry the wafer using a hot plate or oven to eliminate moisture. To reduce the possibility of contamination, wafer preparation is a meticulously regulated and supervised operation that we must do in a cleanroom setting.
Oxidation
The wafer’s surface is then oxidized to form a thin silicon dioxide coating (SiO2). The oxide layer protects the silicon beneath from contamination by acting as an insulator for the IC. The oxidation process is a necessary step in the fabrication of integrated circuits. As a result, a thin layer of oxide forms on the surface of a silicon wafer.
This oxide layer is an insulator and a barrier to prevent undesired impurity penetration into the silicon. Pre-cleaning, dry oxidation, wet oxidation, annealing, surface preparation, and thickness measuring are all part of the oxidation process. Because the quality of the oxide layer is essential to the performance of the integrated circuits created atop it, we monitor the process to ensure that it fulfills tight criteria.
Photoresist Coating
A photoresist is a light-sensitive substance used to produce patterns on a wafer. The photoresist coating process is an essential step in producing integrated circuits. It entails covering the silicon wafer with a photoresist. This photosensitive substance functions as a mask to specify the integrated circuit pattern that we will etch onto the wafer. Next, a spin coater helps to apply the photoresist layer to the wafer’s surface. We then bake it at a low temperature briefly to eliminate any solvents and evaporate any leftover moisture from the coating process.
After exposure, we bake the wafer again at a higher temperature to stabilize the photoresist pattern. Then we immerse the wafer in a developer solution, which dissolves either the exposed or unexposed photoresist depending on the photoresist employed. The process happens in a cleanroom environment to reduce the possibility of contamination. Finally, a spin coating procedure helps to apply the photoresist, which uniformly distributes the material throughout the wafer.
Etching
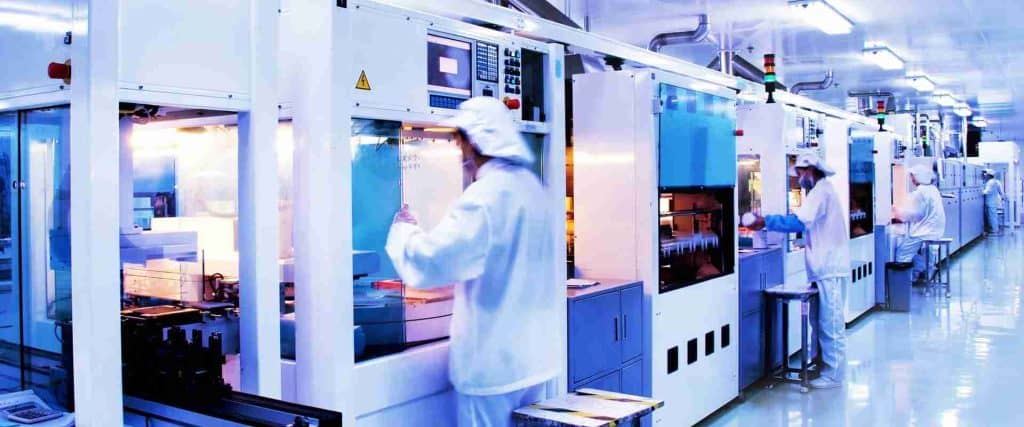
Etching is vital in producing integrated circuits, which help make electronic devices such as microprocessors, memory chips, and sensors. The etching technique includes selectively removing material from a substrate via a chemical or physical reaction.
So, we initially coat the substrate (often silicon) with a layer of photoresist, a light-sensitive substance that hardens when exposed to light. After that, we put a photomask on top of the substrate and beam light through it. Light-exposed portions of the photoresist become hardened, while unexposed parts remain soft.
We subsequently immerse the substrate in an etching solution, eliminating material from the portions not covered by the hardened photoresist. Wet etching, which employs a liquid solution, and dry etching, which uses a plasma, are the two basic etching methods.
After the etching process, we remove the excess photoresist from the substrate using a chemical stripping solution.
We perform this technique several times on the substrate to generate numerous layers of circuitry. Each layer forms using a distinct photomask and etching method, enabling the creation of elaborate circuitry designs on a very small scale.
Doping
Doping is a critical technique in the fabrication of integrated circuits (ICs) that includes the addition of impurities to particular portions of a semiconductor material to affect its electrical characteristics. The doping process aids in forming semiconductor areas with either an excess of electrons (n-type) or an excess of holes (p-type), which are necessary for fabricating different IC components such as transistors, diodes, and capacitors.
Doping typically entails introducing a tiny quantity of dopant atoms into the semiconductor material using one of many processes, including ion implantation, diffusion, or epitaxy. The application and the required electrical characteristics of the resultant semiconductor area determine the dopant utilized.
In n-type doping, for example, atoms with one more valence electron than the semiconductor material, such as phosphorus or arsenic, go into the semiconductor material. These additional electrons are free to move about and contribute to the material’s conductivity, increasing the number of electrons in the semiconductor area.
Similarly, in p-type doping, atoms with one fewer valence electrons than the semiconductor material, such as boron or gallium, go into the semiconductor material. These missing electrons form “holes” in the material, which are positive charge carriers, increasing the number of holes in the semiconductor area.
The doping process is an essential stage in the production of integrated circuits since it allows for synthesizing the components needed for the circuit to work properly. Therefore, careful control of doping amounts and locations is necessary to guarantee that the resultant IC performs consistently and effectively.
Deposition
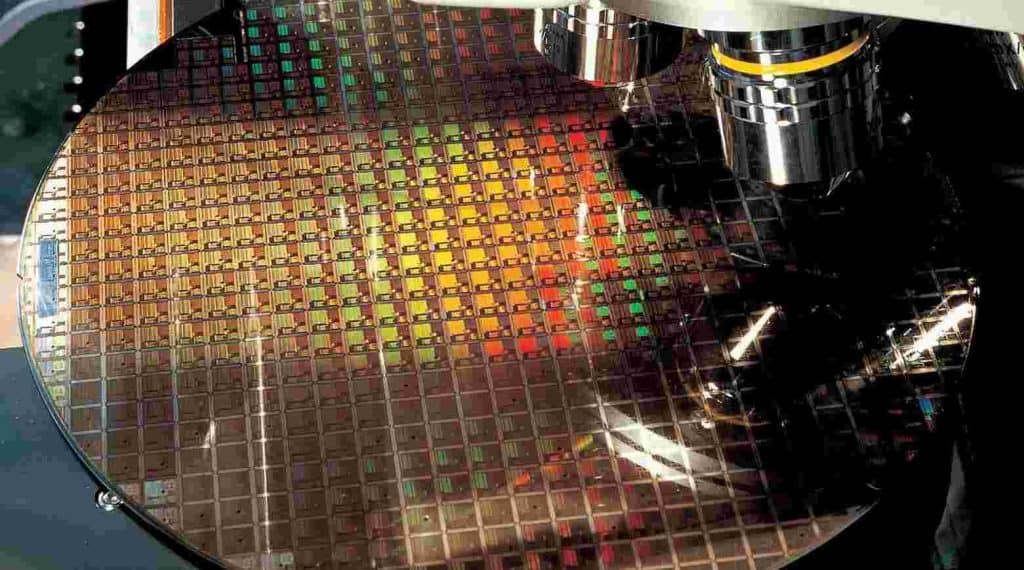
Deposition is a method of depositing thin sheets of material onto a substrate, commonly a silicon wafer, in the fabrication of integrated circuits (ICs). This is a vital phase in manufacturing integrated circuits that involves depositing metals, oxides, and semiconductors. Deposition techniques include physical vapor deposition (PVD), chemical vapor deposition (CVD), and atomic layer deposition (ALD).
PVD deposition involves vaporizing a solid source material in a vacuum and allowing it to condense onto the substrate. The process usually happens in a vacuum chamber, where the substrate heats to a high temperature. Then, we blast the source material with ions or electrons, causing it to evaporate and deposit onto the substrate.
CVD, on the other hand, is the chemical interaction between a gas-phase precursor and the substrate surface that results in the deposition of a material onto the substrate. Then, the precursor gas goes into a reaction chamber. Here, it interacts with the surface of the substrate to generate a thin coating of the desired material.
ALD is a more accurate variant of CVD in which materials are deposited one atomic layer at a time. The technique includes exposing the substrate to two or more precursor gases in succession, depositing one atomic layer of the desired material at a time.
Deposition is an important step in the fabrication of integrated circuits because it dictates the characteristics of the thin films that make up the various layers of the IC. Therefore, we must accurately regulate the thickness, composition, and regularity of these layers to guarantee the appropriate operation of the IC.
Planarization
Chemical mechanical planarization (CMP) is vital in today’s consumer and industrial electronics scene. It offers unparalleled topographical consistency for high-precision electronic wafers, establishing the framework for subsequent etching or deposition procedures.
Silicon (Si) is a good semiconductor material because of its unique electron structure, which allows it to establish perfect covalent connections with other atoms. Silicon’s semiconducting qualities make it excellent for producing diodes, transistors, microelectronics, and other devices. It is the most probable material to usher the electronics industry into the age of systems-on-chips (SOC) and ultra-large-scale integration (ULSI) (ULSI).
Engineers are continually engaging with suppliers to swiftly design innovative solutions that fulfill the demands of device downsizing. We can credit this to rigorous control of silicon wafer planarity down to the sub-nanoscale.
Cleaning and Inspection
Cleaning and inspection are critical steps in the production of integrated circuits. Wet and dry cleaning procedures, optical examination, electron microscopy, and X-ray imaging are Ideal. If we discover them, we rectify defects utilizing several processes, such as laser annealing or chemical etching. When the repairs are complete, we examine the wafers again to confirm that we eliminate all problems. We carefully monitor the process to ensure wafers fulfill stringent quality criteria before manufacturing circuits.
Packaging and Testing
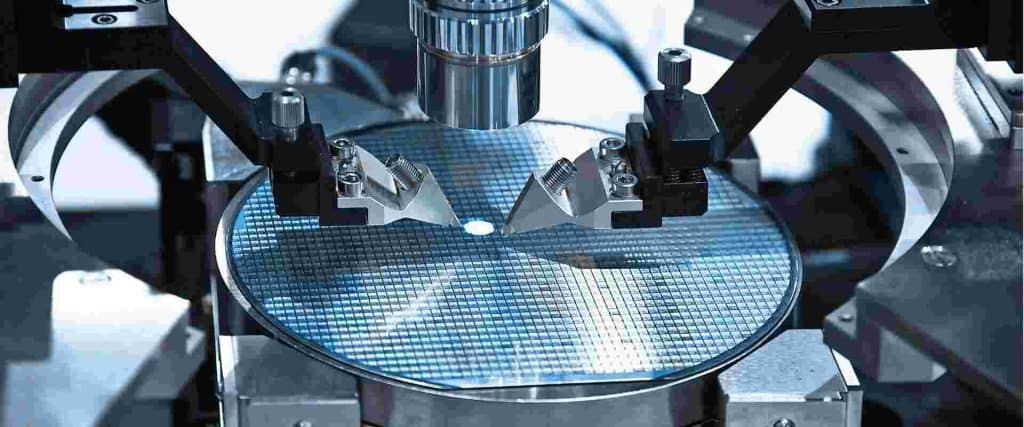
In the production of integrated circuits (ICs), the packaging and testing process is critical. this is because it entails encapsulating and confirming the functionality of the ICs. This procedure is as follows:
Die preparation is the singulating individual dies from a wafer containing several ICs. This is possible by cutting or scribing the wafer along preset lines, separating each die from the wafer.
Die attachment: Using a die-bonding machine, the individual die bonds to a lead frame or a substrate at this point. This machine employs conductive adhesive.
Wire bonding: use a wire-bonding machine to link the die to the lead frame. The wire-bonding machine uses ultrasonic energy to connect a tiny wire from the bonding pad on the die to the lead frame.
Encapsulation: We enclose the IC in a protective container after wire bonding to protect it from external elements such as moisture, dust, and mechanical damage. Encapsulation materials are generally thermoset plastics or ceramics.
Testing: When encapsulating the IC, we subject it to a series of tests to confirm its functioning. Functional testing, performance testing, and reliability testing are all possibilities. These tests guarantee that the integrated circuit fulfills the specified requirements and standards.
Marking and sorting: After passing all tests, we label the IC with a component number, lot number, and date code. We then sort the integrated circuit depending on its speed, temperature range, and packaging type properties.
Final inspection: We scrutinize the IC for faults like scratches, cracks, or other physical problems at the final inspection step. This will guarantee that the IC satisfies the quality criteria necessary.
Conclusion
Finally, due to its unique electrical characteristics, silicon is the most extensively helpful material for constructing integrated circuits. Silicon is a semiconductor, meaning it may conduct electricity under certain conditions. Additionally, we can adjust its conductivity by introducing impurities. Silicon is also extremely stable and corrosion-resistant, making it a perfect material for use in electrical equipment.