A printed circuit board (PCB) is a non-conductive substrate used to mechanically support and electronically connect electronic components using conductive pathways or traces etched from copper sheets laminated onto the substrate. PCBs provide the foundation for almost all modern electronic devices and enable the physical implementation, connectivity and packaging of circuit designs.
This article provides a comprehensive overview of PCB technology, manufacturing processes, materials, design considerations and applications across various industries.
PCB Basics
A PCB consists of multiple layers of insulating and conducting materials pressed together and then etched into specific electrical circuit layouts designed to implement complex electronic systems in a small form factor.
Key elements of a PCB include:
- Substrate material – commonly FR-4 glass epoxy or other insulating material
- Conductive layers – copper foils laminated on substrate to create signal traces
- Pads/lands – widened areas of copper traces for soldering components
- Plated through holes (PTHs) – plated barrel interconnects between layers
- Solder mask – insulating layer covering exposed copper from oxidation and short circuits
- Silkscreen – printed component outlines and identifiers
PCBs provide a consistent platform for assembly of electronic components and integration into complete functioning systems. The etched copper traces replace individual wires, enabling denser, higher reliability connections.
PCB Manufacturing Process Overview
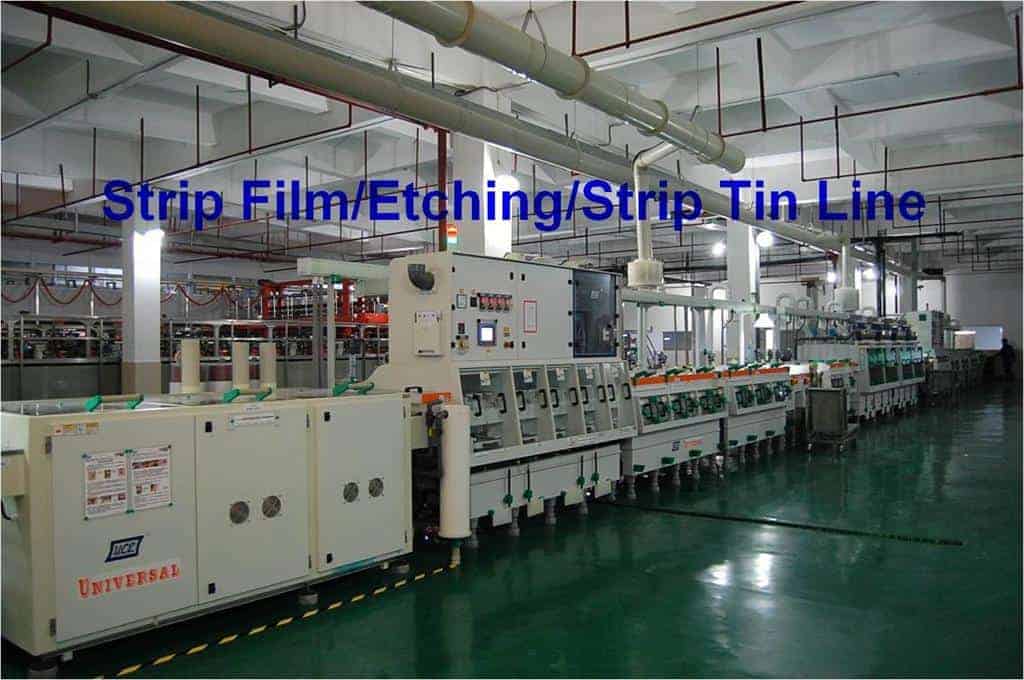
PCBs are fabricated using sophisticated processes optimized for high yield and reliability across large panel sizes containing multiple boards:
1. Design – The PCB layout is designed in CAD software based on circuit requirements and component footprints.
2. Fabrication – Blank copper-clad laminate sheets are drilled, plated and coated with photoresist. An imaging process transfers the PCB layout onto the panel.
3. Etching – Exposed copper is etched away chemically, leaving only the protected circuit traces/pads. Photoresist is then stripped.
4. Plating – Conductive barrel walls are plated in drilled holes to create plated through hole (PTH) interconnections.
5. Solder mask – Epoxy layers are applied for insulating exposed copper traces from solder and environmental corrosion.
6. Silkscreen – Paint masks are used to apply component identifiers and outlines for assembly.
7. Testing – Manufacturing defects are detected using electrical test and imaging processes.
8. Panelization – Individual PCBs are depanelized from the larger sheets into stand-alone boards.
9. Population – Electronic components are soldered onto the boards using surface mount (SMT) and/or through-hole technologies.
These steps produce finished printed circuit boards ready for integration into electronic products and systems.
PCB Substrate Materials
The PCB substrate, also referred to as laminate, forms the base layer upon which the copper traces are fabricated. Key laminate requirements include:
- Electrical insulation to prevent shorting between traces
- Thermal conductivity to dissipate heat from components
- Structural rigidity for mechanical support
- Dimensional stability across temperature and humidity
- Process compatibility for fabrication and assembly
Popular PCB substrate materials include:
- FR-4 – Woven fiberglass cloth in an epoxy resin binder. Low cost, globally available.
- CEM-1/3 – Cotton paper base material. Cost effective, suitable for low frequencies.
- PTFE – Synthetic fluoropolymer offering highest performance but costly.
- Polyimide – Withstands very high temperatures. Used in flex circuits.
- Alumina – Ceramic-based; very rigid and thermally conductive for high power PCBs.
- Metal core – Base metal layer for max heat dissipation.
The substrate choice affects PCB performance parameters, fabrication methods and ultimate application suitability.
Conductive Layers
The conducting layers provide the electrical connectivity in a PCB by etching the laminated copper foils into desired circuit patterns. Key considerations include:
- Copper thickness – Varies from 0.5 oz (0.0007 inches) to 6 oz (0.0042 inches) or more based on current needs.
- Foil type – Rolled copper has better flexibility but lower current capacity than electrodeposited copper.
- Layer count – Number of conductive layers laminated determines circuit complexity. Modern PCBs can have over 30 conductive layers.
- Layer stackup – Stacking order of power, ground and signal layers optimized for electrical performance.
- Trace dimensions – Conductor width, spacing and thickness dictated by current, voltages and insulation needs.
- Trace profile – Rectangular or trapezoidal cross-section optimized for production yields.
Proper layer stackup design and copper weight/density minimizes noise coupling and enables routing high speed signals across the PCB.
Plated Through Holes
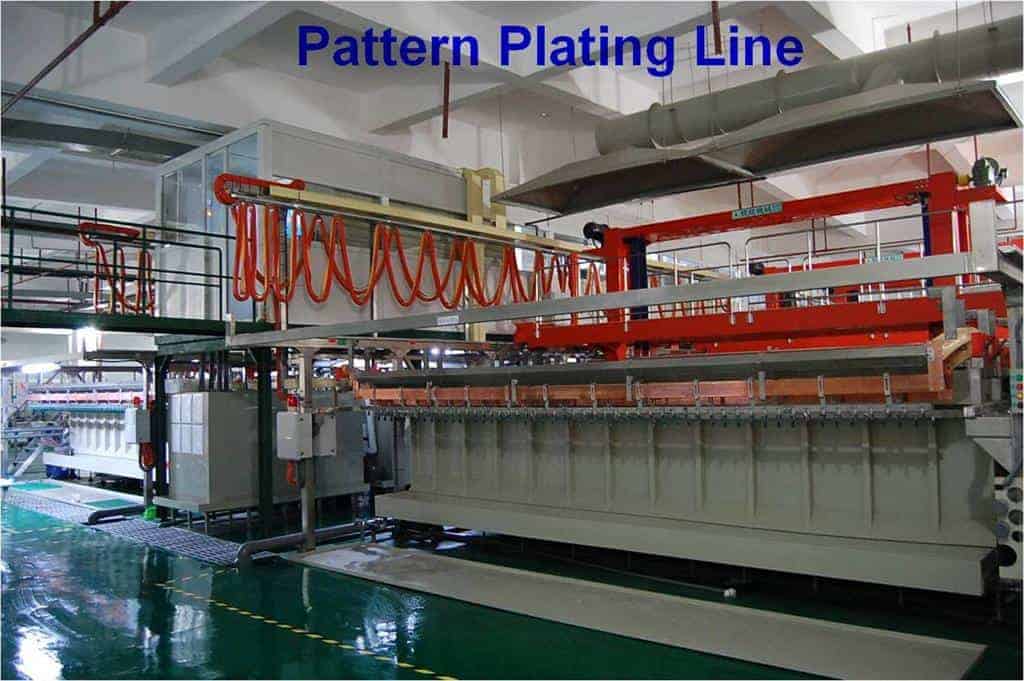
Plated through holes (PTHs) are conductive barrels fabricated by drilling holes through the PCB layers and plating the internal walls to form electrical connections between layers. Benefits include:
- Provides vertical interconnects through entire PCB thickness
- Connects traces on different layers for routing signals
- Connects internal power/ground planes for power distribution
- Enables component leads to pass through board and solder on opposite side
- Higher reliability and current capacity than vias
PTHs are essential for multilayer PCB construction, power delivery, attaching connectors and through-hole component soldering.
PCB Fabrication Methods
There are several common techniques to fabricate printed circuit boards:
Subtractive Process
The subtractive process starts with a copper clad laminate consisting of substrate fully coated with copper on one or both sides. PCB fabrication involves:
- Drilling holes for vias/PTHs
- Photoresist application and lithographic imaging to transfer layout patters
- Etching to remove unwanted copper leaving only intended copper traces
- Photoresist stripping
- Plating copper barrels in drilled holes to form PTHs
Subtractive processes are suitable for high volume PCB production achieving fine features down to 5 mil trace/space.
Additive Process
The additive process fabricates conductive traces by adding copper only in the desired areas through electroplating:
- Drill holes for vias/PTHs in blank laminate
- Plate copper in drilled holes to form PTHs
- Photoresist dispensing on areas without circuit traces
- Electrodepositing copper in openings to build up traces
- Photoresist removal once plating completed
Additive processing is more complex but generates less chemical waste. High density interconnect (HDI) PCBs leverage this process.
Semi-Additive Process
Semi-additive combines aspects of additive and subtractive methods:
- Starting with copper clad laminate, drill holes and plate PTH barrels
- Blanket electroless plate thin copper seed layer
- Lithographic patterning and copper electroplating to build up traces
- Etching away seed layer copper from unplated areas
Semi-additive technique provides a compromise between waste generation and process complexity.
PCB Design and Layout
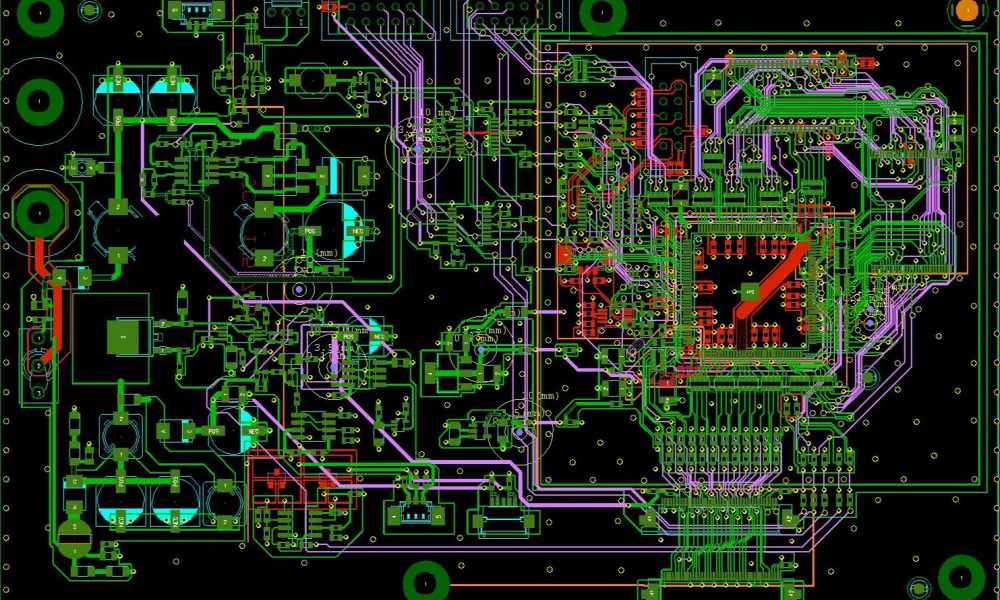
PCB layout involves arranging interconnects between electronic components in a physical manner that optimizes electrical performance, manufacturability, costs, reliability and other factors.
Key aspects of PCB layout include:
- Component footprints – Land/pad geometries matching component lead configurations
- Net routing – Interconnects between component pins implemented as traces on layers
- Thermal management – Strategic placement and copper fills to dissipate heat
- EMI/EMC controls – Minimizing interference and susceptibility
- Signal integrity – Tuned trace impedance, spacing, rise times for quality signal propagation
- Manufacturing rules – Accommodating tolerances, annular rings, fabrication constraints
- Test points – Providing access points for validation and troubleshooting
- Assembly considerations – Ease of component placement, orientation and soldering
Following sound PCB design practices ensures the layout can be accurately produced within budget.
PCB Design Software
Modern PCBs are designed using electronic design automation (EDA) tools which enable:
- Circuit schematic capture
- Linkage between schematics and layout
- Footprint generation and component libraries
- Constraint-driven signal and power routing
- 3D visualization
- Design rule checking
- Output of manufacturing files like Gerber
Popular PCB design software includes Altium Designer, Cadence Allegro, Mentor Xpedition, and Cadence OrCAD.
PCB Assembly and Soldering
Once fabricated, a bare PCB is populated with electronic components to form a functional printed circuit assembly (PCA). The two main methods are:
Through-Hole Assembly
- Component leads are inserted through holes in PCB body
- Leads soldered to barrel walls of plated through holes
- Provides strong mechanical connection
Surface Mount Assembly
- Components directly soldered to pads on PCB surface
- Enables miniaturization and higher density
- Reflow soldering heats entire board for mass solder joint formation
- Mixed SMT and through-hole assembly also widely used
For high volume production, SMT lines utilize pick-and-place machines and conveyorized reflow. Rework stations allow modifying soldered assemblies.
PCB Classifications
PCBs can be classified based on the board complexities:
Class 1 – Single sided PCB with components on one side only
Class 2 – Double sided PCB with components on both sides
Class 3 – Multilayer board with components on outer layers only
Class 4 – Multilayer board with components on both sides and internally
More complex multilayer PCBs enable higher component densities and more optimized electrical layouts. Common modern applications utilize minimum 4 layer (2 signal + 2 power/ground) boards.
Key PCB Characteristics
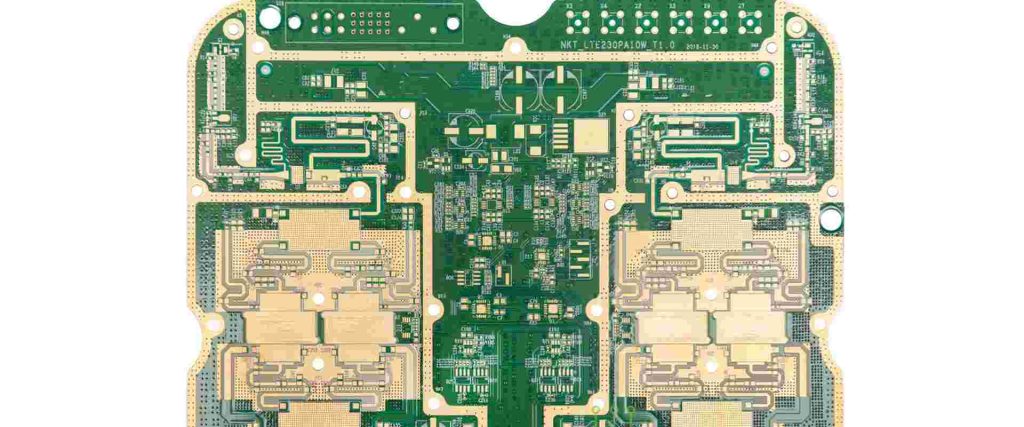
Essential characteristics defining a PCB’s capabilities:
- Number of conductive layers – Represents PCB complexity; more layers provide higher component density
- Laminate material – Determines electrical, thermal, mechanical characteristics
- Trace/space – Narrowest conductor width and gap; 5 mil line/space common in commercial PCBs
- Finished copper thickness – After plating; affects current capacity and manufacturability
- Microvias – Small vias connecting adjacent layers; enables greater vertical connectivity
- Hole size – Smallest drilled hole diameter; smaller holes provide higher interconnect density
- L/S Ratio – Ratio of maximum layer count to minimum trace/space
PCB Classification and Grades
IPC, the Association Connecting Electronics Industries, has established standards for classifying PCBs based on quality levels:
Class 1 – General purpose PCBs with basic electrical test and quality conformance inspection.
Class 2 – PCBs requiring more controlled material and fabrication criteria with electrical testing. Used for routers, servers, switches, industrial controls.
Class 3 – High reliability PCBs manufactured under tightly controlled conditions and fully electrically tested. Used in enterprise network hardware, telecom infrastructure.
Class 4 – Mission critical PCBs for aerospace, high-end computing. Entails exhaustive product conformance and testing.
Stringent standards for lamination, drilling and imaging enables Class 3 and Class 4 PCBs to be produced with very low defect rates.
PCB Cost Drivers
Key factors impacting overall PCB fabrication costs:
- Board size – Overall area determines panel utilization efficiency
- Layer count – Additional layers require more process steps and materials
- Finer features – Narrower traces/spaces need advanced capabilities
- Hole sizes/PTH density – More and smaller holes increase drill costs
- Higher tolerances – Holding tighter tolerances adds process overhead
- Panel utilization – Packing efficiency impacts cost allocation
- Laminate material – Exotic substrates cost more than standard FR-4
- Lead times – Rush fabrication has higher costs
- Order volumes – High volume production affords better economy of scale
PCB Design for Manufacturing Guidelines
Adhering to design for manufacturing (DFM) principles enhances PCB fabrication yields and minimizes costs:
- Use minimum trace widths/spaces permissible for currents/voltages
- Avoid using multiple different trace widths; standardize sizes
- Minimize excessive voids requiring complex fill polygons
- Plan layout for efficient board break optimization
- Allow sufficient clearance around pads and traces for solder mask overlap
- Limit high aspect ratio of traces that can cause etching issues
- Use 90 or 45 degree angles rather than arcs for traces
- Avoid traces between closely spaced pads which are hard to etch reliably
Considering fabrication practicalities during layout avoids unnecessary cost overruns and delivery delays.
PCB Types and Form Factors
PCBs support a diverse array of electronics applications through specialized constructions:
Rigid PCBs – Most widely used; made of rigid laminate substrate
Flex PCBs – Use flexible polyimide or polyester base materials
Rigid-Flex PCBs – Combine rigid sections for components and connectors with flexible interface sections
HDI PCBs – High density interconnects with microvias for compact stacking
Multi-Chip Modules – Miniature substrates embedding bare ICs for high density
Aluminum Backed PCBs – Bonded to a base aluminum plate for thermal spreading
High Frequency PCBs – Using specialized materials engineered for optimal RF/microwave response
High Power PCBs – With thicker copper and/or dielectric and thermal layers to sustain higher currents and heat dissipation
PCB Design Software
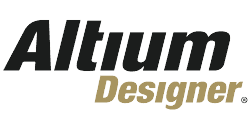
PCBs are designed using sophisticated CAD software which provides:
- Schematic capture with linkage to layout
- Intelligent part/footprint libraries
- Constraint-driven automated routing
- Visual preview of designs in 3D
- Design rule checks to ensure manufacturability
- Output of Gerber files for fabrication
- Modeling tools for signal and power integrity analysis
Leading vendors include Altium, Cadence, Mentor Graphics, and Zuken.
Key PCB Technology Trends
- Continued miniaturization enabling higher component densities and thinner PCBs.
- More board layers (20+ layers) allowing complex circuit partitioning.
- Microvias under 0.15mm in diameter providing high interconnect density.
- Flexible substrates facilitating rollable and shape-conforming PCBs.
- Embedded actives and passives for further size reduction and improved electrical performance.
- Direct imaging replacing photochemical methods for lower costs and waste.
- Higher throughput equipment such as mass lamination presses.
- PCB fabrication directly on thin silicon substrates for advanced packaging.
- Laser-based micromachining for high precision drilling and structuring.
- Conductive composites replacing copper for specialized applications.
PCB Reliability and Testing
Ensuring reliable PCB performance across product lifetimes requires robust design and qualification:
Design Analysis
- Thermal modeling to optimize heat dissipation and prevent overheating
- Vibration modeling to determine mechanical resonance risks
- Signal integrity analysis through IBIS models to prevent coupling
Qualification Testing
- Temperature cycling exposes boards to thermal extremes
- Power cycling evaluates performance under hot operating conditions
- Vibration testing checks for risks of fracture or component failure
- Bend testing for flex boards validate stable operation through repeated flexing
- HAST (highly accelerated stress testing) provides rapid lifetime wearout indication
- Shear, pull and torque testing checks solder joint integrity
Test Methods
- In-circuit testers validate component functionality and solder connections on populated boards
- Flying probe testers perform bare board testing through mechanical probes
- Boundary scan tests board wiring and connections between components
- Automated optical inspection looks for physical defects
- X-ray imaging reveals hidden defects and flaws
Robust qualification testing and design analysis improves overall PCB quality and long term reliability in the field.
PCB Applications Across Industries
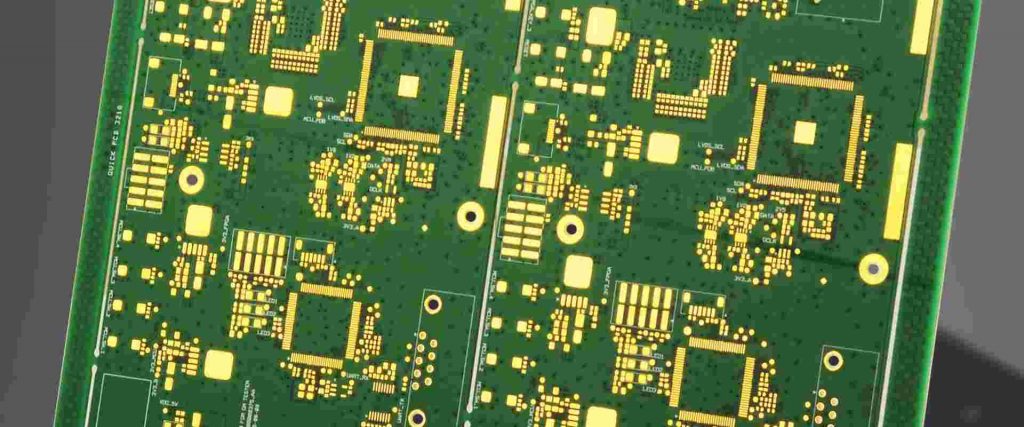
PCBs provide the foundation for electronics across every industry:
Consumer Electronics – Smartphones, laptops, tablets, wearables, home appliances, gaming consoles.
Automotive – Engine control units, dashboards, in-vehicle infotainment, LiDAR/RADAR components.
Aerospace/Defense – Avionics electronics, navigation, communications, weapon systems.
Computing – Servers, networking switches, data storage systems.
Communications – 5G and optical infrastructure, baseband processing, routing/switching.
Medical – Implants, diagnostic imaging systems, patient monitors, clinical lab instruments.
Industrial – Programmable Logic Controllers (PLCs), robotics, motor drives, process control electronics.
Virtually every electrical system across every industry leverages PCB technology to efficiently interconnect components and encapsulate complex circuits in compact, robust and cost-effective formats.
Conclusion
PCB fabrication represents the foundation of electronics, enabling the physical implementation of circuit schematics through precise copper pathways etched on insulating substrates. Over decades, PCB technology has evolved enormously – from simple single-sided boards to multilayer high density interconnects with thousands of components.
PCBs have facilitated exponential growth in electronics innovation and permeated every industry. Understanding PCB materials, manufacturing processes, design considerations and applications provides key insight into the most ubiquitous of electronics building blocks. Ongoing advances in fabrication techniques, packaging approaches, interconnect densities, component embedding and thermal management will ensure PCBs remain at the heart of electronics systems for decades to come.
Frequently Asked Questions
Q: What is a printed circuit board?
A: A PCB is a non-conductive substrate with printed copper pathways to interconnect electronic components as per the circuit design topology.