Introduction
Micro-electromechanical systems, or MEMS, are tiny integrated devices that combine mechanical and electrical components on a common silicon substrate. MEMS extend the fabrication techniques developed for the integrated circuit industry to add mechanical elements like beams, gears, diaphragms, and springs to chips.
In this comprehensive guide, we will cover:
- The components and operating principles of MEMS devices
- Various types of MEMS sensors, actuators, and other applications
- MEMS materials, manufacturing processes, and packaging
- Design and simulation considerations for MEMS
- Current and future applications using MEMS
- MEMS industry landscape and key players
- Advantages and challenges with MEMS technology
- FAQs
By the end, you’ll have a solid grasp of what micro-electromechanical systems are, how they work, where they are used, and their impact. Let’s get started!
Components and Operating Principles of MEMS
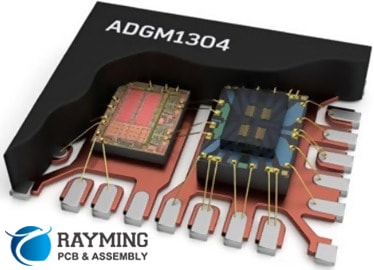
Micro-electromechanical systems integrate miniature:
- Sensors – To detect physical phenomena like motion, pressure, chemical levels, etc. Common examples include accelerometers, gyroscopes, pressure sensors, and gas sensors.
- Actuators – To provide physical movement or action. Examples include micromirror arrays, valves, pumps, and manipulators.
- Electronics – To process signals from sensors and control actuators. The circuitry includes components like ASICs, capacitors, transistors, and bond pads.
- Mechanical Structures – The micromachined components that enable physical functionality like cantilevers, gears, membranes, channels, and diaphragms.
By combining these miniaturized elements on a chip, MEMS enable new categories of tiny sensors, actuated devices, and integrated systems not achievable with conventional electronics.
MEMS operate through principles like:
- Thermal actuation
- Piezoelectric transduction
- Capacitive sensing
- Electrostatic actuation
- Resonant energy harvesting
- Microfluidic control
These enable functions difficult to implement conventionally at such small scales. Cutting edge fabrication techniques make MEMS possible.
Types of MEMS Devices
There are several major categories of MEMS devices:
MEMS Sensors
Sensors detect physical phenomena and convert them into electronic signals for processing.
Accelerometers – For measuring acceleration and tilt along 1, 2 or 3 axes. Critical for airbags, phones, navigation, vibrations, and more.
Gyroscopes – Measure angular velocity and orientation in space. Used in drones, VR/AR, robotics, and other applications needing precision orientation.
Pressure Sensors – Gauge applied pressure. Used in weather monitoring, touchscreens, biomedical devices, and more.
Chemical Sensors – Detect gaseous chemicals and concentrations. Enable smart gas detection and analysis.
Inertial Measurement Units – Combine accelerometers and gyroscopes to precisely track motion and orientation for navigation and guidance.
Resonant Sensors – Vibrating MEMS structures shift frequency based on mass or other changes, allowing extremely precise measurements.
MEMS Actuators
Actuators provide movement or mechanical action. Examples include:
Micromirror Arrays – Control light beams by tilting tiny mirrors for displays, scanning, and fiber optics.
Microvalves – Regulate fluid flows in miniature channels and pipes. Used in miniature gas chromatography systems, lab-on-a-chip devices, etc.
Optical Switching – Route optical signals between fiber optic channels. Used in telecommunications and data networking equipment.
RF MEMS Switches – Switch high frequency electrical signals for tuned impedance matching and signal routing. Used in tunable filters, phased arrays, and reconfigurable antennas.
Microspeakers – Vibrate to generate sound waves and audio output. Used in earphones and integrated device sound.
MEMS Pumps – Generate controlled microfluidic flows or pressure differentials. Used for drug delivery, analyte sampling, microcooling systems, etc.
Other Types of MEMS
Beyond sensors and actuators, other types of MEMS include:
- Microfuel cells – Generate electricity from fuel sources through electrochemical reactions on a chip.
- Micromotors – Spin tiny rotors using electromagnetic forces or thermal actuation. Used for micro gears, pumps, and other mechanisms.
- Microbolometers – Measure infrared radiation for thermal imaging applications. Used in fire detection, autonomous vehicles, and security cameras.
- Microneedle patches – Contain arrays of microneedles for drug delivery through the skin.
The diversity of MEMS devices enables sophisticated miniature systems across a huge range of applications, as we’ll explore next.
MEMS Materials and Manufacturing Processes
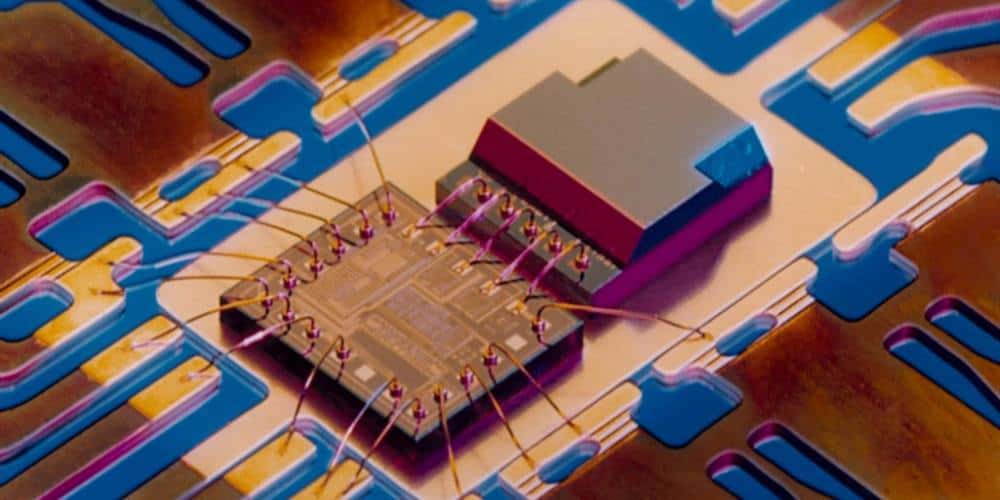
Fabricating MEMS requires specialized processes, equipment, and materials. Key aspects include:
Substrate Materials
- Silicon is the most common substrate material due to well-controlled microfabrication techniques developed by the IC industry.
- Other options include glass, ceramics, metals, and polymers.
Deposition Processes
- Depositing thin films of structural and sacrificial materials through processes like chemical vapor deposition (CVD), sputtering, and molecular beam epitaxy.
Lithography
- Patterning masks and exposing photoresists using techniques like photolithography, e-beam lithography, and X-ray lithography.
Etching
- Removing material selectively through wet etching with acid solutions or dry etching using plasma.
Doping
- Ion implantation and diffusion to dope structural layers like polysilicon with boron, phosphorus or other dopants.
Bonding
- Anodic bonding of micromachined silicon wafers with glass layers.
Micromachining
- Bulk micromachining to selectively etch inside silicon or other substrates.
- Surface micromachining to build structures by patterning layers on top of the substrate.
Careful sequencing of these microfabrication steps allows constructing the mechanical and electrical components needed for MEMS.
MEMS Packaging
Once fabricated, MEMS dies require specialized packaging to:
- Protect delicate MEMS structures from damage
- Facilitate required gas or fluid flows
- Allow space for movements
- Electrically connect bond pads
- Mount components like application-specific integrated circuits (ASICs)
- Enable integration into larger systems
Common packaging approaches include:
- Ceramic and plastic packaging
- Wafer-level packaging and encapsulation
- Multi-chip module packaging
- Printed circuit board integration
Innovations in MEMS packaging continue advancing capabilities while reducing size and cost.
MEMS Design and Simulation
Designing MEMS requires cross-functional physics simulation and modeling tools. Key analyses performed include:
- Mechanical – Stress, vibration, fatigue life, resilience, etc.
- Electrical – Drive circuitry, feedback signals, noise, etc.
- Thermal – Heat dissipation, thermal lags, conduction, etc.
- Fluidic – Air/liquid flows, pressure differentials, turbulence, etc.
- Optical – Light reflection, diffraction, scattering, etc.
Each MEMS application has unique multiphysics requirements that must be modeled across mechanical, electrical, fluidic, and thermal domains synergetically.
Standard 3D CAD tools combined with multiphysics solvers address MEMS modeling needs for precision design optimization.
Current and Future Applications of MEMS Technology
Thanks to their tiny size, low cost, and sophisticated functionality, MEMS devices are enabling innovative applications across every industry:
Transportation – Inertial guidance, ESP, safety sensors in airbags, tire pressure monitoring, etc.
Consumer Electronics – Accelerometers, gyroscopes, microphones, and displays in smartphones, game consoles, etc.
Medical – MEMS pressure sensors in catheters, microneedle drug delivery, implants, diagnostics.
Industrial – MEMS accelerometers for vibration monitoring, gas sensors for leak detection, microvalves for control.
Defense – MEMS inertial measurement units in missiles and drones, chemical threat detection, research into MEMS robots and smart dust.
Scientific – Lab-on-a-chip devices, microanalysis systems, portable microsensors.
Communications – RF MEMS switches for tuned antennas, optical routing, phase array transmitters.
Auto – Tire pressure sensors, inertial sensors, infrared detectors for night vision systems.
The next wave of innovation in self-driving vehicles, IoT devices, AR/VR, Industry 4.0, and more will be enabled by MEMS. The opportunities are boundless thanks to the unique capabilities MEMS provide.
MEMS Industry Landscape
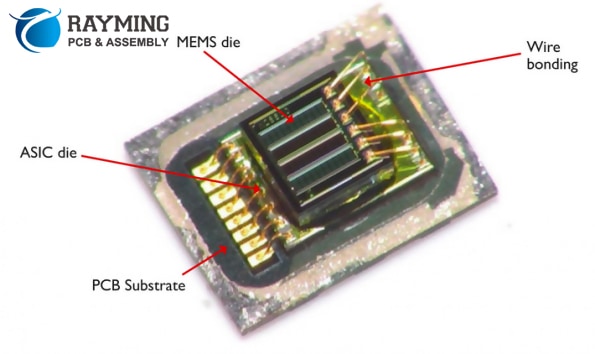
The global MEMS industry is projected to grow to around $90 billion by 2025. Key players include:
- Semiconductor foundries like TSMC, GlobalFoundries, and SMIC that fabricate MEMS devices.
- Fabless MEMS designers including STMicroelectronics, Bosch Sensortec, InvenSense, and mCube.
- IDMs with internal MEMS manufacturing like Texas Instruments and Analog Devices.
- Applied Materials, SUSS MicroTec, and DISCO providing fabrication equipment.
- OSATs packaging and testing MEMS including ASE, Amkor, and JCET.
- Automotive Tier 1s producing MEMS sensors for vehicles, such as Denso, Delphi, and Infineon.
While initially driven by a small group of IDMs, today’s MEMS industry involves an advanced supply chain enabling high volume foundry production. Economies of scale continue driving increased adoption.
Advantages and Challenges of MEMS
Some key benefits MEMS provide versus conventional implementations:
Benefits
- Extreme miniaturization impossible through other means
- Fully integrated devices combining electronics and mechanical elements
- Low power consumption due to reduced size
- Cost effective high-volume fabrication
- Performance improvements from scaling effects
Challenges
- High development costs and long design cycles
- Need for advanced modeling and simulation skills
- Strict process controls and expertise required
- Packaging for protection while allowing operation
- Metrology of 3D microscopic features
By capitalizing on their advantages while navigating inherent challenges, MEMS stand to revolutionize nearly every industry much like ICs did for electronics.
Frequently Asked Questions
Here are some common MEMS-related questions:
Q: How small are MEMS devices typically?
A: MEMS range from 20 micrometers to 1 millimeter. Very small yet large enough to allow practical microfabrication. State-of-art processes steadily allow smaller MEMS over time.
Q: How are MEMS different from ICs?
A: While fabricated similarly to ICs, MEMS add physical micromechanical structures rather than just electronic circuits. This allows mechanical sensing and actuation.
Q: Are MEMS always made from silicon?
A: Most use silicon substrates due to readily available silicon micromachining techniques from the IC industry. But some MEMS leverage materials like polymers.
Q: How durable and reliable are MEMS components?
A: MEMS can last decades in many applications with careful design. However, certain environments with high temperatures, pressures, radiation or contaminants can impact reliability.
Q: Are MEMS expensive to produce?
A: MEMS fabrication has high upfront costs for masks and process development. However, the per unit costs become very affordable in high volume thanks to batch manufacturing principles.
Conclusion
In summary, micro-electromechanical systems fuse electrical and mechanical components on integrated chips to enable transformative applications. With ongoing innovations in manufacturing and design, MEMS will continue growing as a key technology across industries from automotive to medical to consumer electronics and more. Their unique advantages of microscopic size, integration, low cost, and sophisticated performance open up new possibilities. While engineering MEMS requires specialized expertise, the benefits make the investment well worth it for next-generation products and capabilities.