Power Factor Correction (PFC) is a crucial component in modern power supply designs, ensuring efficient and clean power delivery. One of the key aspects of PFC design is understanding and measuring the current loop, which plays a vital role in the overall performance and reliability of the system. In this comprehensive article, we will delve into the intricacies of measuring the current loop of a PFC, exploring various techniques and considerations.
Understanding the PFC Current Loop
Before diving into the measurement techniques, it’s essential to understand the PFC current loop and its significance. The PFC current loop consists of the input rectifier, the PFC choke (or inductor), the PFC switch (typically a MOSFET), and the associated circuitry. This loop carries the high-frequency switching currents, which can reach substantial levels, especially in higher-power applications.
The current loop is critical for several reasons:
- EMI (Electromagnetic Interference): The high-frequency switching currents in the PFC current loop can generate electromagnetic interference, which can affect the operation of nearby electronic devices or even the power supply itself. Proper management and measurement of the current loop are essential for EMI mitigation.
- Power Losses: The current loop carries substantial currents, which can lead to significant power losses due to resistive heating and stray inductances. Measuring the current loop helps identify and minimize these losses, improving overall efficiency.
- Reliability: High currents and associated losses can cause thermal stress on components, potentially leading to premature failure or reduced lifespan. Accurate measurement of the current loop aids in thermal management and ensures reliable operation.
- Compliance: Many regulatory bodies impose strict guidelines and standards regarding EMI and power quality. Measuring the current loop is crucial for ensuring compliance with these regulations.
Measurement Techniques
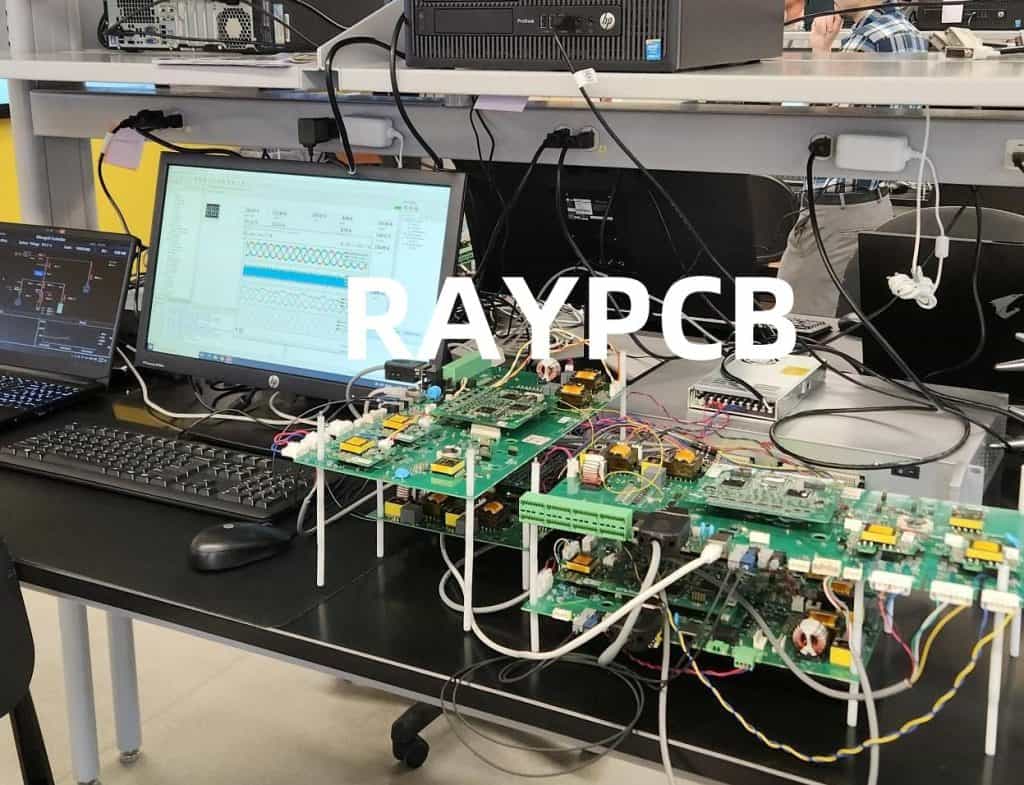
There are several techniques available for measuring the current loop of a PFC. Each method has its advantages and drawbacks, and the choice depends on factors such as accuracy requirements, cost, and ease of implementation.
1. Current Probe
One of the most common and straightforward methods for measuring the current loop is using a current probe. Current probes are non-invasive devices that clamp around the current-carrying conductor and measure the magnetic field generated by the flowing current.
Current probes come in various types, including:
- AC Current Probes: These probes are designed to measure alternating currents and are suitable for high-frequency applications like PFC current loops.
- DC Current Probes: While primarily intended for measuring DC currents, some DC current probes can also handle high-frequency AC signals, making them suitable for PFC current loop measurements.
- Current Transformer (CT): Current transformers are similar to current probes but are designed for higher currents and often used in power distribution systems. They can also be used for PFC current loop measurements, although their frequency response may be limited.
When using current probes, it’s essential to consider factors such as frequency response, bandwidth, and accuracy specifications to ensure reliable measurements.
2. Resistive Shunt
Another widely used technique for measuring the current loop is the resistive shunt method. In this approach, a low-value, high-precision resistor is inserted in series with the current path being measured. The voltage drop across this shunt resistor is proportional to the current flowing through it, allowing for current calculation based on Ohm’s law.
Resistive shunts offer several advantages:
- High accuracy: With careful selection and calibration, resistive shunts can provide highly accurate current measurements.
- Wide bandwidth: Properly designed shunts can handle a wide range of frequencies, making them suitable for PFC current loop measurements.
- Cost-effective: Resistive shunts are generally less expensive than other measurement techniques, especially for low-to-medium current ranges.
However, resistive shunts also have some drawbacks:
- Voltage drop: The shunt resistor introduces a voltage drop in the current path, which may not be desirable in some applications.
- Power dissipation: The shunt resistor dissipates power, which can lead to heating and potential thermal management issues, especially at higher currents.
- Invasive: Inserting a shunt resistor requires breaking the current path, which may not be feasible or desirable in some designs.
3. Current Sensing Amplifiers
Current sensing amplifiers, also known as current shunt amplifiers or current shunt monitors, combine the principles of the resistive shunt method with amplification and signal conditioning circuitry. These devices incorporate a precision resistor and amplifier in a single package, providing an amplified and isolated voltage output proportional to the measured current.
Current sensing amplifiers offer several advantages:
- Isolation: The amplifier circuit provides galvanic isolation between the current path and the measurement circuitry, enhancing safety and reducing noise.
- Low voltage drop: The amplifier allows the use of a lower-value shunt resistor, reducing the voltage drop in the current path.
- Integrated solution: Many current sensing amplifiers are available as integrated circuits, simplifying design and reducing board space requirements.
However, current sensing amplifiers also have some limitations:
- Cost: While integrated solutions offer cost savings compared to discrete designs, current sensing amplifiers can be more expensive than simple resistive shunts.
- Bandwidth limitations: Some current sensing amplifiers may have limited bandwidth, which could impact their performance in high-frequency PFC current loop measurements.
4. Rogowski Coil
The Rogowski coil is a unique current measurement technique that relies on the principle of mutual inductance. It consists of a toroidal coil of wire, typically wrapped around the current-carrying conductor without making an electrical connection.
When current flows through the conductor, it induces a voltage in the Rogowski coil proportional to the rate of change of the current (di/dt). This induced voltage can be integrated to obtain the current waveform.
Rogowski coils offer several advantages:
- Non-invasive: Since the Rogowski coil does not make electrical contact with the current path, it is a non-invasive measurement technique.
- Wide bandwidth: Rogowski coils can provide accurate measurements over a wide range of frequencies, making them suitable for PFC current loop measurements.
- Flexibility: Rogowski coils can be easily wrapped around conductors of various sizes and shapes, providing flexibility in measurement setups.
However, Rogowski coils also have some limitations:
- Integration circuit complexity: The induced voltage from the Rogowski coil needs to be integrated to obtain the current waveform, requiring additional circuitry or digital signal processing.
- Position sensitivity: The accuracy of Rogowski coil measurements can be affected by the positioning and orientation of the coil around the conductor.
- Cost: High-quality Rogowski coils and associated integration circuitry can be relatively expensive compared to other measurement techniques.
5. Hall Effect Sensors
Hall effect sensors are another non-invasive method for measuring currents. These sensors rely on the Hall effect principle, where a voltage is generated across a conductive material (typically a semiconductor) when it is exposed to a magnetic field perpendicular to the current flow.
In the context of PFC current loop measurements, Hall effect sensors can be placed near the current-carrying conductor, allowing for non-invasive current measurement based on the magnetic field generated by the flowing current.
Hall effect sensors offer several advantages:
- Non-invasive: Like Rogowski coils, Hall effect sensors do not require direct electrical contact with the current path, making them non-invasive.
- Galvanic isolation: Hall effect sensors provide inherent galvanic isolation between the measurement circuitry and the current path, enhancing safety and reducing noise.
- Wide bandwidth: Many Hall effect sensors can operate over a wide range of frequencies, making them suitable for PFC current loop measurements.
However, Hall effect sensors also have some drawbacks:
- Sensitivity to external fields: Hall effect sensors can be susceptible to interference from external magnetic fields, which can affect measurement accuracy.
- Linearity and offset errors: Hall effect sensors may exhibit non-linearities and offset errors, which require careful calibration and compensation.
- Temperature sensitivity: The performance of Hall effect sensors can be affected by temperature variations, requiring temperature compensation or stable operating conditions.
Considerations and Best Practices
When measuring the current loop of a PFC, there are several important considerations and best practices to keep in mind:
- Bandwidth and Frequency Response: Ensure that the chosen measurement technique has sufficient bandwidth and frequency response to accurately capture the high-frequency switching currents present in the PFC current loop.
- Probe Positioning: For current probes, Rogowski coils, and Hall effect sensors, proper positioning and orientation around the current-carrying conductor are crucial for accurate measurements. Follow the manufacturer’s guidelines and best practices.
- Ground Loops and Noise: Proper grounding and shielding practices are essential to minimize the impact of ground loops and external noise sources on current loop measurements.