The age of long GPS navigators has lapsed, thanks to rapid technological advances in the area of electronics. As a result, you cannot rely on the large GPS navigators to assist with direction like your grandfathers did years back. Instead, it has become simpler to incorporate GPS capabilities into your printed circuit board. Therefore, getting a consumer electronic device with a GPS PCB antenna has never proved more straightforward.
However, if you lack the RF design or GPS experience, you can toil when designing an integrated GPS PCB product. So how can you go about it? We will delve into that in a bit but first, let us delve into what a GPS PCB entails.
GPS PCB: What It Entails
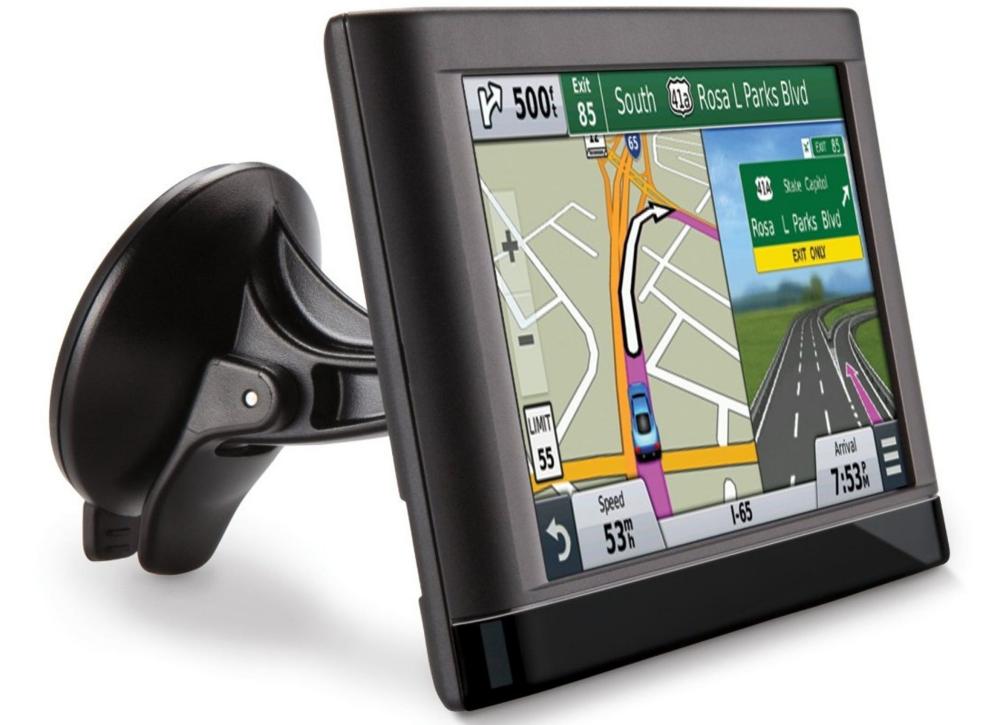
A GPS circuit board entails a small printed circuit board equipped with a GPS module like most circuit boards. The GPS module can encompass a GNSS antenna, a ceramic patch, a GPS patch antenna, etc. As a GPS PCB designer, you must consider both the GPS antenna aspects and the printed circuit board aspects you desire. Your intended application area also plays a crucial role. However, one of the critical aspects of a GPS entails its antenna. It defines the type of GPS PCBs to a large extent.
Active and Passive GPS Antenna
You will probably find GPS PCB antennas as either two types, passive or active antennas. The difference between the two encompasses an active antenna with an LNA (low-noise amplifier) while a passive antenna lacks one. An active antenna sits on its board and connects to your PCB (printed circuit board) through a coax cable.
You will find some receivers coming prepackaged with one of either type antenna types. Additionally, it can also possess a passive matching network that matches a 50 Ohms impedance pattern of radiation output. However, an active antenna possesses a performance advantage because its LAN maintains the noise level in the output signal. Such noise often results in a higher sensitivity.
Passive antennas on printed circuit boards also need to deploy an LNA, though the signal quality degrades as the signal travels to the LNA from the receiver. Any extra noise limits its general sensitivity since an LNA by design needs to reduce the output signal’s noise. Further, whenever you choose to deploy a receiver needing an external LNA, the signal trace that runs to the LNA has to be shielded from crosstalk or external EMI the best you can.
GPS Antenna in Your Printed Circuit Board Design
It is prudent to know that utilizing or incorporating a GPS antenna in your printed circuit board transitions it into a regime of mixed signals. Every noise introduced at the input antenna from crosstalk or EMI degrades the signal quality. It can also wholly block the antenna signal besides making it susceptible to ground plane noise, especially when not adequately isolated from surrounding components.
Other components located on your printed circuit board need proper isolation; otherwise, the GPS receiver and antenna can degrade the signal in such components. You will realize that the receiver can prove the worst noise culprit in most instances, primarily when the receiver possesses an internal antenna. Additionally, the crosstalk or EMI between other components and the receiver underscores the importance of including proper shielding.
You have to include filtering so that you can extract the GPS signal. One of the primary ways of achieving this entails placing a SAW (surface acoustic wave) filter between the receiver input and the LNA. A SAW filter allows for the filtering or sifting of high frequencies, mostly above 1 GHz. It gets found in GPS-based applications. It is unheard of to extract a GPS frequency from surrounding noise in the signal minus a SAW filter.
Grounding, Shielding, and Routing
The output signal from a GPS receiver/antenna often proves below the noise floor with around 30 dB. As such, acceptable minor noise in some applications could easily block signals from the GPS receiver. It would help to have a proper grounding, shielding, and routing for the GPS-enabled device’s proper function.
In most instances, diving your primary printed circuit board into functional blocks implies also providing each with its ground plane. After that, the ground planes need to get routed back to the primary ground lead (mostly in star topology) to avert ground loops. However, the enormous requirements when it comes to size for a ground plane that surrounds a GPS receiver make it difficult, primarily in mobile gadgets or devices.
However, if you shield your receiver, any external LNA, and its matching network within a shielding can, connecting the digital ground planes and RF becomes possible. All you need to do entails isolating the GPS receiver besides matching the network to its RF ground plane. You then connect it to the digital ground plane on one point. The ideal place to ground the data lines and clock should be the RF ground plane.
Antenna traces that run to the receiver carry analog signals and get placed far from the digital components and traces. If possible, you can route the antenna traces within a shielded enclave. It is possible to bury the antenna traces within an internal printed circuit board layer besides placing the matching circuit’s ground planes on either side. The embedded antenna needs to get positioned outside the shield. However, try to shield the battery and other electronics from the antenna.
Impedance Matching Design
As an RF or high-frequency designer, you understand that impedance and attenuation matching proves crucial in contributing to the degradation of the signal. Often, higher carrier frequency signals possess longer trace results and more significant attenuation, especially in low overall sensitivity. Therefore, always go for a shorter trace between your external LNA and your passive receiver/antenna. It helps in keeping the sensitivity high.
It would help to avoid vias when running antenna traces carrying the RF signals. Such vias enhance the trace’s impedance by creating an inductive discontinuity. Such a discontinuity adds an approximate impedance of 10 Ohms to your trace (for GPS RF frequencies). Further, vias having a larger diameter adds a more significant impedance. For instance, if your antenna/ receiver already has a passive impedance of 50 Ohms, it becomes prudent to compensate for the vias appearing in the trace.
Before contacting us at RayMing PCB and Assembly for your GPS tracker PCB fabrication or assembly, you can always use layout software such as Altium Designer to incorporate a GPS antenna into your PCB design. The software has an comprehensive range of tools to make your PCB layout flourish. For instance, you can use smart design rule checking, simulation and analysis tools, and auto-interactive routing potential to ensure a flawless design. Such a design then becomes simpler to transition into the prototyping and production phases.
Design Factors to Consider in Choosing a GPS Module
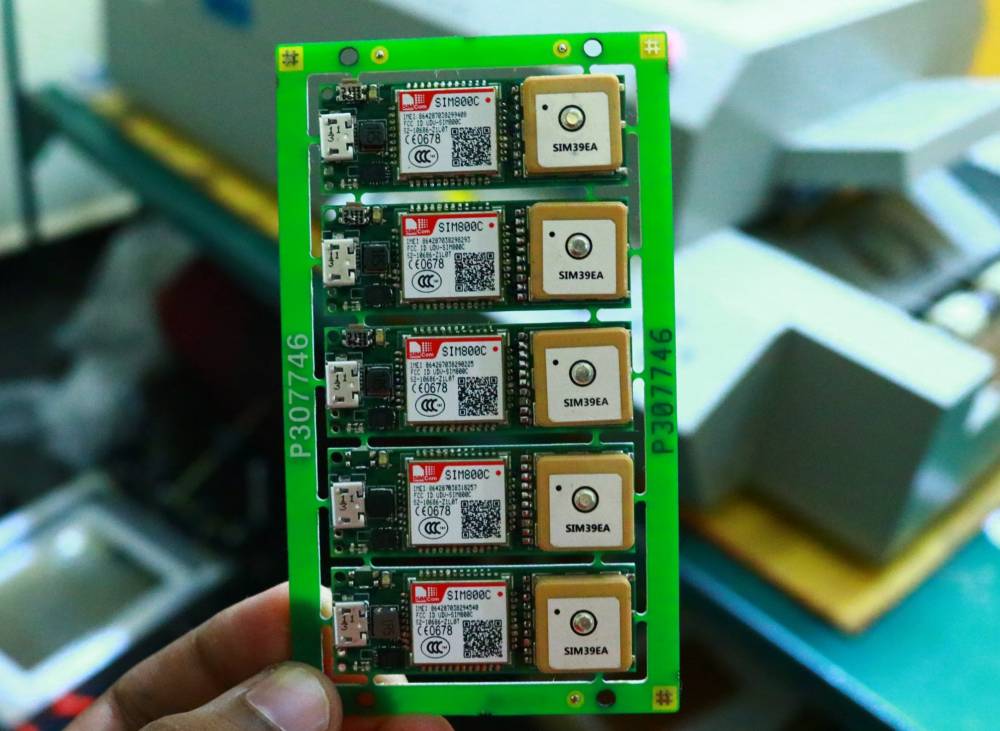
GPS chipsets and modules have become integral for industrial and consumer devices in the current dispensation. Such demand has increased the emphasis of GPS to every PCB engineer or designer. After all, the onus falls on them to incorporate the GPS subsystem into the printed circuit board designs.
However, not every printed circuit board designer or engineer has experience with GPS PCB design. Therefore, it becomes helpful to consider the following design rules to successfully integrate the GPS receiver into the PCB design.
Considerations
You have to pick a GPS module or chipset as the first step. Additionally, it would help to consider other factors before choosing a GPS solution and before embarking on the design process.
Can you pay for a GPS simulator when it comes to testing and fabrication?
The costs of GPS simulators can prove prohibitive based on the type. For instance, single-channel simulators go for around $10,000 to $15,000. You, therefore, need to account for such a cost in your PCB product. If you cannot, a GPS module proves a better idea for your GPS PCB system. However, you will still have to design the 50-Ω RF traces or tracks despite the module coming qualified and pretested. Therefore, the BOM cost for this alternative will prove steeper.
While the temptation to use a GPS repeater or do away with the antenna can cross your mind owing to the associated costs, either option tends to result in poor-test coverage. You, therefore, stand a better chance of designing a better-quality GPS PCB for production tests by using a GPS simulator.
Is the goal of the GPS antenna passive or active?
You have learned of the benefit of using an active antenna. One standout aspect entails the built-in LNA (low-noise amplifier) that connects to your printed circuit board via a coax cable. On the other hand, a passive antenna lacks an LNA in their antenna element and thus have to get mounted onto the printed circuit board.
A passive antenna design proves more complex than prone to noise coupling (on the antenna ground plane) when not appropriately isolated from other components that produce noise on the printed circuit board. You can also face challenges with testing passive antenna designs because a GPS simulator, re-radiating antenna, and an FR chamber typically require a setup and a calibration for proper function (consistent testing results).
What antenna element type do you want?
Antenna element types come in all manner of shades and can prove linear, patch, chip, etc. You have to determine what you need before the design phase kicks in. for instance, the typical patch antenna within the range of 15-25mm, coupled with a 40-mm ground plane, provides the best performance, especially in mobile equipment. However, it may prove too large for your specific application, which then forces you to go for tinier antenna topologies like a chip or linear antennas.
It is critical to remember when deciding between patch and chip antennas. A patch antenna can give you the best signal to its size. It arises because a patch antenna receives signals on either side of the patch. On the other hand, a linear GPS (dipole or chip) will only get signals along a single axis. It thus results in linear antennas proving less sensitive by about a half (3 dB) than patch antennas. Most linear antennas have about 25% sensitivity to a patch antenna.
A few of the latest folded-F and chip designs show promise in the area of sensitivity. First, however, it always becomes essential to assess your GPS sensitivity requirements. Here, you can use your top evaluation kit or a module manufacturer utilizing diverse antenna topologies in determining your best fit.
However, if you do not prefer designing an antenna, you can opt for a patch antenna or an active chip from RayMing PCB and Assembly. Such a unit gives you a tested module besides allowing you, as the designer, to implement a GPS simulator via a U.FL connector.
What Follows Next After Deciding on a GPS Chipset?
Once you have settled on a GPS chipset of your choice besides the antenna topology, the next step entails some research before getting dirty with the actual design process. First, it helps if you understand some basics of GPS signals and their signal strength, for starters. When it comes to signals, the maximum GPS transmission comes to 160 dB or 130 dBm. Such a full signal strength translates to 20 dB below the RF noise floor of the receiver/antenna.
Spectrum analyzers, besides other standard RF equipment, cannot detect this signal. In most cases, the GPS antenna or receiver (its RF front-end) fails to have a traceable, capturable, and probe-capable analog signal. You can, therefore, only detect the GPS signal’s presence by correlating with the GPS receiver. It all implies that all the GPS performance and testing metrics have to involve the GPS receiver-generated signal data, which acts as a pivotal aspect of the test procedure.
Getting Started with the Design
In most cases, you will potentially want to copy the reference GPS PCB design you have come across your GPS chipset company or vendor. However, understanding that the GPS signal ranks lower than the noise floor can come a long way. You must take and run away with a concept if you want to become successful in your design process.
When considering a GPS receiver, a simple design that passes as “quiet” on the EMC (electromagnetic compatibility) test might not prove so quiet. Plenty of digital noise around the globe makes it difficult, and thus the first step would entail isolation. While it may not offer much reprieve as far as a noise-free design gets concerned, it should provide a decent start on trying to achieve it.
When it comes to the ground plane, isolating the GPS RF in front of the receiver section becomes necessary. You then connect it to its RF ground plane that connects the digital section at one point. It also represents the preferred section to connect the data lines and the clock. What’s more, you also have to regulate the flow of current out and into the GPS section.
Normally, in-line resistors located in the data paths and clock can regulate the current flow. It minimizes the rapid current spikes common with a change of signal state. In your design considerations, you also have to remember that the ground connection acts as the return path for transferable energy within the signal path.
Additionally, tracking the power supply into the GPS section becomes necessary, especially on the single traces. It becomes crucial because placing the ground and power planes on top of each other generates a plate capacitor, whereas the printed circuit board material acts as the dielectric.
How do you deal with the noise?
Any noise generated on the power plane needs to get coupled into the ground plane, having noisy results. It is also prudent to approach the rest of the digital section the same way because some of the noise can permeate to the GPS RF section, primarily through the SPGC (single-point ground connection)
Strip Line, Trace Impedance, and Via Control
You have to run the RF, ground-return, and digital traces once you finish placing the components and have isolated and defined your ground planes. Further, you have to get your impedance set at 50 Ω. It, therefore, implies that the need for an impedance-controlled printed circuit board becomes unnecessary.
If your printed circuit board manufacturer gives you the freedom to set the plane-to-plane spacing, then you can regulate it yourself. If you get an FR4-based printed circuit board material, you will probably get the trace width as a function of the spacing of the layers.
You can always calculate the trace width, though this often proves complex to most upcoming PCB designers. However, with plenty of the online tools available nowadays, you can always calculate it online. However, one of the fundamental values you will still have to calculate entails the PCB permeability value, as you need this for the trace width calculation. In most instances, a value in the range of 4.3 – 4.7 works, though an FR4 trace width value of 4.5 works as well in a few cases. However, always consult with your printed circuit board manufacturer to verify this before proceeding to the next stage.
The trace thickness generally falls between 35 µm (for copper measuring 1-oz) and 17 µm for copper weighing 0.5-oz. The 0.5 oz copper typically applies to the inner layers. You also have to consider the impact of the vias on the trace’s impedance. For instance, at GPS frequencies, ever via doubles up as tiny inductor. As a result, each via will add approximately 10 Ω of trace impedance. The number of traces used in this case has a multiplier effect on the impedance mismatch.
How to Resolve the Design Issues
Always keep the RF traces on the uppermost or top PCB layer. The RF grounds that do not connect to the top layer’s ground return path need additional (multiple) vias. You have to keep in mind that reducing inductance implies increasing the number of inductors in parallel. Therefore, the power grounds like the decouplers (decoupling caps) must get implemented with individual vias. However, decoupling components needs proximity to the component, especially those with a direct connection.
It also takes 0.9 inches, trace length, to develop an FR4-based antenna element (0.25-wave) at GPS frequencies. You also have to place components in an end-to-end fashion besides avoiding track-stubs.
Crucial Components of a GPS PCB Receiver
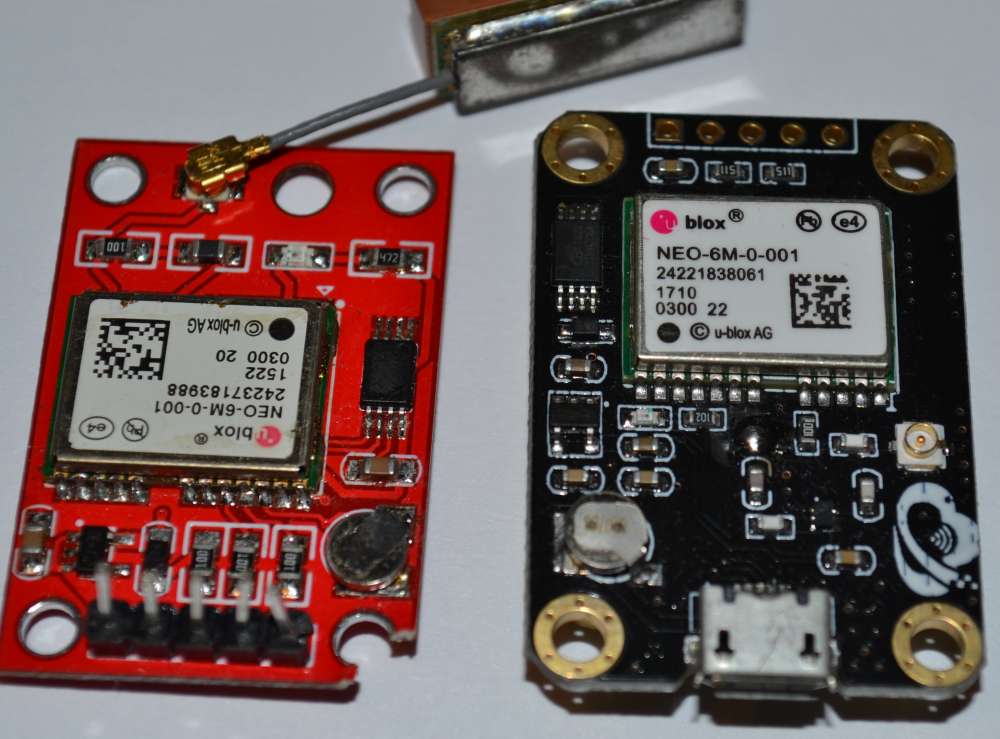
LNA, which represents the initial stage of the GPS receiver, requires a low-noise power supply for it to function correctly. The best way to guarantee minimal noise entails providing the RF with its LDO or low-dropout regulator. Most of the available LDOs possessing the noise rejection feature range from 50 – 70 dB. Further, it comes affordable as it will only cost you thirty cents when in large volumes. It also becomes necessary for you to incorporate a cap and inductor (noise regulation) between the RF supply and the LNA if not featured in your design reference. It safeguards the LNA from voltage-controlled oscillator (VCO) noise within the RF.
Additionally, SAW filters have also become important in a lot of environments. All you need to do here entails following the matching components guide from your reference design. You can also ask for matching details where you get your SAW filter. However, remember to safeguard the ground connections through the SAW filter body.
It is important to remember the TCXO requirement for TTFF (fast time-to-first-fix) and contains an original tolerance minimum of 2.5 ppm. But for GPS application or operations, the oscillators have to prove ultra-stable within the one-Hz time domain. However, the TCXO needs protection from quickly changing thermal transients (a shield). You can always search for and work with a professional GPS TCXO solutions provider. Remember, a general-purpose TCXO cannot suffice in this scenario.
You also have to place heat-producing components outside the shield. Such components include power transistors, voltage regulators, etc. Similarly, place the components that produce noise like high-speed oscillators, switching regulators, and quickly switching circuits outside the shield as well.
A Common Misnomer
It so happens that most UHF RF and VHF shielding connect all the points of the shield can to the printed circuit board’s ground plane. It, therefore, can prove a mistake with GPS frequencies as its signal’s open-air wavelengths prove shorter than the UHF. Furthermore, based on the shield can’s size, current can flow through it, making it resonate near the GPS frequencies. Consequently, it interferes or causes de-tuning of the GPS-RF.
You can avoid this occurrence by creating a shielding ring that links to the shield can and connects to the RF ground via an inductor. It is the inductors that will filter EMI-induced current flow.
Final Thoughts
GPS PCBs have become essential elements in the sea, air, and land navigation. Understanding its design and importance in the contemporary world helps make your design better and improve it in the process. Hopefully, throughout this piece, you have answered some of the nagging questions about GPS PCB design.